Researchers have shed insight on the molecular mechanisms driving hibernation, with their findings published today as a Reviewed Preprint in eLife.
The editors regard their research in small and big hibernating mammals as an important work that advances our understanding of the function of myosin structure and energy use in the molecular mechanisms of hibernation, supported by strong methodology and data. The findings also imply that myosin, a kind of motor protein involved in muscular contraction, has a role in non-shivering thermogenesis during hibernation, in which heat is created regardless of muscle activity (shivering).
Hibernation is a survival strategy utilized by many species, characterized by deep dormancy and significant decreases in metabolic activity, body temperature, heart rate, and respiration. During hibernation, animals rely on stored energy stores, namely lipids, to keep their bodies functioning. The metabolic slowdown enables hibernators to conserve energy while enduring long periods of food scarcity and harsh climatic circumstances in the winter. However, the underlying cellular and molecular mechanisms of hibernation remain poorly known.
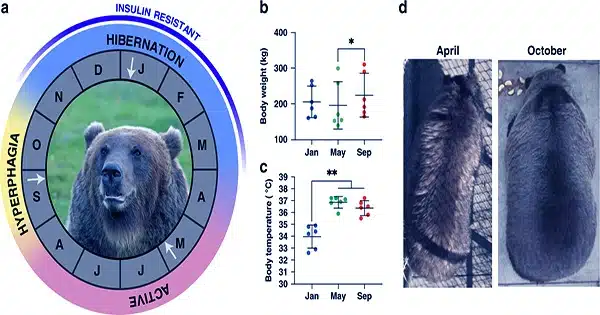
Smaller hibernating mammals go through extended periods of torpor, a hypo-metabolic state that significantly lowers their body temperature and is punctuated by spontaneous interbout euthermic arousals (IBA), in which they temporarily raise their body temperature to restore some physiological functions, such as waste elimination and food consumption.
In contrast, larger mammals’ body temperatures remain relatively constant during hibernation. Skeletal muscle, which accounts for around half of a mammal’s body mass, is critical in determining heat generation and energy expenditure.
“Until recently, energy consumption in skeletal muscles was assumed to be principally tied to the activity of myosin, a protein involved in muscular contraction. However, there is accumulating evidence that skeletal muscles require a tiny amount of energy even when they are relaxed,” says main author Christopher Lewis, a postdoctoral researcher at the University of Copenhagen’s Department of Biomedical Sciences in Denmark.
“In passive muscles, myosin heads can be in two resting states: ‘disordered-relaxed,’ or DRX, and super-relaxed,’ or SRX.” Myosin heads in the DRX state consume ATP—the cell’s energy currency—between five and ten times faster than those in the SRX state,” Lewis says.
Lewis and colleagues postulated that variations in the amount of myosin in the DRX or SRX states could contribute to the lower energy use observed during hibernation. To test this, they collected skeletal muscle samples from two small hibernators, the thirteen-lined ground squirrel and the garden dormouse, as well as two large hibernators, the American black bear and the brown bear.
First, they investigated if myosin states and ATP consumption rates differed between active and hibernating periods. They examined muscle fibers collected from the two bear species during their active summer phase (SA) and winter hibernation periods.
The fraction of myosin in the DRX or SRX states was found to be identical between the two stages. They employed a specific technique known as the Mant-ATP chase assay to determine myosin’s rate of ATP consumption. This also demonstrated that myosin’s energy consumption rates remained unchanged. This could be to prevent considerable muscle atrophy in bears during hibernation.
The scientists also performed the Mant-ATP chase assay on small animal samples collected during SA, IBA, and torpor. They found no variations in the percentage of myosin heads in SRX or DRX creation between the three phases, just as they did in larger hibernators. However, they discovered that the ATP turnover time of myosin molecules in both forms was shorter in IBA and torpor than in the SA phase, resulting in an unanticipated overall increase in ATP consumption.
Small mammals have a greater drop in body temperature during hibernation than large mammals, thus the team investigated if this surprising rise in ATP use happened at a lower temperature. They repeated the Mant-ATP chase test at 8°C instead of the previously used ambient lab temperature of 20°C. Lowering the temperature reduced DRX and SRX-linked ATP turnover times in SA and IBA, resulting in increased ATP consumption.
Metabolic organs, such as skeletal muscle, are well known for increasing core body temperature in response to prolonged cold exposure, either by shivering or non-shivering thermogenesis. Cold exposure increased ATP consumption by myosin in samples collected during SA and IBA, implying that myosin may contribute to non-shivering thermogenesis in tiny hibernators.
The scientists found no cold-induced alterations in myosin energy use in samples taken during torpor. They believe that this is a protective mechanism for maintaining the low core body temperature and wider metabolic shutdown observed during torpor.
Finally, the researchers wanted to know what modifications occurred at the protein level during the various hibernation periods. They investigated whether hibernation alters the structure of two myosin proteins found in the thirteen-lined ground squirrel, Myh7 and Myh2. Although they found no hibernation-related alterations in the structure of Myh7, they discovered that Myh2 underwent considerable phosphorylation—a step critical for energy storage—during torpor, as opposed to SA and IBA.
The scientists found no cold-induced alterations in myosin energy use in samples taken during torpor. They believe that this is a protective mechanism for maintaining the low core body temperature and wider metabolic shutdown observed during torpor.
Finally, the researchers wanted to know what modifications occurred at the protein level during the various hibernation periods. They investigated whether hibernation alters the structure of two myosin proteins found in the thirteen-lined ground squirrel, Myh7 and Myh2. Although they found no hibernation-related alterations in the structure of Myh7, they discovered that Myh2 underwent considerable phosphorylation—a step critical for energy storage—during torpor, as opposed to SA and IBA.
They also examined the two proteins in the brown bear and discovered no structural variations between SA and hibernation. They conclude that Myh2 hyperphosphorylation is exclusively related to torpor, rather than hibernation in general, and hypothesize that this serves to promote myosin stability in small mammals. This could serve as a molecular mechanism to reduce myosin-associated increases in skeletal muscle expenditure in response to cold exposure during times of torpor.
The editors of eLife highlight that some aspects of the study require further investigation. Muscle samples were collected solely from the legs of the animals investigated. Given the temperature differences between the core body and the limbs, studying muscle samples from various parts of the body would help to corroborate the team’s findings.
“Overall, our findings indicate that ATP turnover adaptations in DRX and SRX myosin states occur in tiny animals like as the thirteen-lined ground squirrel during cold-weather hibernation. In contrast, larger mammals such as the American black bear exhibit no such alterations, most likely due to their steady body temperature during hibernation,” says principal author Julien Ochala, Associate Professor at the University of Copenhagen’s Department of Biomedical Sciences. “Our results also suggest that myosin may act as a contributor to skeletal muscle non-shivering thermogenesis during hibernation.”