Quantum science is a rapidly changing field with ongoing research, and scientists are constantly developing new tools and techniques to investigate and comprehend quantum phenomena. Researchers created a ‘quantum ruler’ to measure and investigate the unusual properties of multilayered sheets of graphene, a type of carbon. The research could also result in a new, miniaturized electrical resistance standard that could be used to calibrate electronic devices directly on the factory floor, eliminating the need to send them to an off-site standards laboratory.
Graphene, a single-atom-thick sheet of carbon, has remarkable properties on its own, but things get even more interesting when multiple sheets are stacked together. When two or more graphene sheets are slightly misaligned – twisted at certain angles relative to each other – they assume a variety of exotic identities. Depending on the twist angle, these moiré quantum matter materials can generate their own magnetic fields, become superconductors with zero electrical resistance, or become perfect insulators.
Joseph A. Stroscio and his colleagues at the National Institute of Standards and Technology (NIST), in collaboration with an international team of collaborators, have created a “quantum ruler” to measure and investigate the strange properties of these twisted materials. The research could also result in a new, miniaturized electrical resistance standard that could be used to calibrate electronic devices directly on the factory floor, eliminating the need to send them to an off-site standards laboratory.
Using the new quantum ruler to study how the circular orbits vary with magnetic field, we hope to reveal the subtle magnetic properties of these moiré quantum materials.
Joseph A. Stroscio
Collaborator Fereshte Ghahari, a physicist from George Mason University in Fairfax, Virginia, took two layers of graphene (known as bilayer graphene) of about 20 micrometers across and twisted them relative to another two layers to create a moiré quantum matter device. Ghahari made the device using the nanofabrication facility at NIST’s Center for Nanoscale Science and Technology.
The twisted material device was then chilled to one-hundredth of a degree above absolute zero by NIST researchers Marlou Slot and Yulia Maximenko, reducing random motions of atoms and electrons and increasing the ability of electrons in the material to interact. After reaching ultralow temperatures, they investigated how the energy levels of electrons in graphene layers changed as the strength of a strong external magnetic field was varied. The ability to measure and manipulate electron energy levels is critical for designing and manufacturing semiconductor devices.
To measure the energy levels, the team used a versatile scanning tunneling microscope that Stroscio designed and built at NIST. When the researchers applied a voltage to the graphene bilayers in the magnetic field, the microscope recorded the tiny current from the electrons that “tunneled” out from the material to the microscope probe tip.
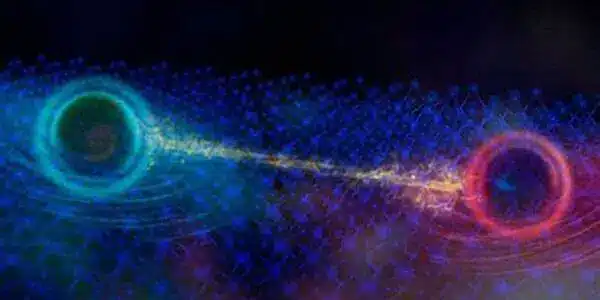
Electrons move in circular paths in a magnetic field. Normally, the circular orbits of electrons in solid materials have a special relationship with a magnetic field applied: Due to the quantum nature of electrons, the area enclosed by each circular orbit multiplied by the applied field can only take on a set of fixed, discrete values. If the magnetic field is halved, the area enclosed by an orbiting electron must double in order to maintain that fixed product. The difference in energy between successive energy levels that follow this pattern can be used to measure the material’s electronic and magnetic properties, much like tick marks on a ruler. Any subtle deviation from this pattern would represent a new quantum ruler that can reflect the orbital magnetic properties of the particular quantum moiré material researchers are studying.
In fact, the NIST researchers discovered evidence of a new quantum ruler in action when they varied the magnetic field applied to the moiré graphene bilayers. The area enclosed by an electron’s circular orbit multiplied by the magnetic field applied no longer equaled a fixed value. Instead, the product of those two numbers had shifted by an amount determined by the bilayer magnetization.
This deviation was translated into a series of different tick marks for the electrons’ energy levels. The findings could shed new light on how electrons trapped in twisted sheets of graphene produce new magnetic properties.
“Using the new quantum ruler to study how the circular orbits vary with magnetic field, we hope to reveal the subtle magnetic properties of these moiré quantum materials,” Stroscio said.
In moiré quantum materials, electrons have a range of possible energies – highs and lows, shaped like an egg carton — that are determined by the electric field of the materials. The electrons are concentrated in the lower energy states, or valleys, of the carton. The large spacing between the valleys in the bilayers, bigger than the atomic spacing in any single layer of graphene or multiple layers that aren’t twisted, accounts for some of the unusual magnetic properties the team found, said NIST theoretical physicist Paul Haney.
The researchers published their findings in the journal Science, along with colleagues from the University of Maryland in College Park and the Joint Quantum Institute, a research collaboration between NIST and the University of Maryland.
The new measurements promise to provide a deeper understanding of how scientists can tailor and optimize the magnetic and electronic properties of quantum materials for a variety of applications in microelectronics and related fields because the properties of moiré quantum matter can be chosen by selecting a specific twist angle and number of atomically thin layers. Ultrathin superconductors, for example, are already known to be exquisitely sensitive detectors of single photons, and quantum moiré superconductors are among the thinnest.
The NIST team is also interested in another application: moiré quantum matter may provide a new, simpler standard for electrical resistance under the right conditions.
The current standard is based on the discrete resistance values assumed by a material when a strong magnetic field is applied to electrons in a two-dimensional layer. The quantum Hall effect is caused by the same quantized energy levels as the electrons in the circular orbits discussed above. To calibrate the resistance in various electrical devices, discrete resistance values can be used. However, because a strong magnetic field is required, calibrations can only be performed at a metrology facility such as NIST.
Stroscio believes that if researchers can manipulate quantum moiré matter so that it has a net magnetization even in the absence of an externally applied magnetic field, it could be used to create a new portable version of the most precise resistance standard, known as the anomalous quantum Hall resistance standard. Electronic device calibrations could be performed on-site, potentially saving millions of dollars.