The size of black holes varies. At the heart of most nearby, big galaxies is considered to be a supermassive black hole (hundreds of thousands to billions of times the mass of our Sun). Other black holes, far smaller in size, are collapsed stars. Light cannot escape from black holes because they are so dense. This means we cannot “see” them directly, but there are indirect ways to detect and learn about them.
The Universe is teeming with energetic particles including X rays, gamma rays, and neutrinos. However, the sources of the majority of high-energy cosmic particles remain unknown. An interdisciplinary scientific team has now presented a theory that explains these phenomena: low-activity black holes operate as significant producers of high-energy cosmic particles. Details of their research were published in the journal Nature Communications.
Gamma rays are very intense photons that are many orders of magnitude more powerful than visible light. Cosmic gamma rays with energy ranging from megaelectron to gigaelectron volts have been discovered by space satellites. Neutrinos are subatomic particles with near-zero mass. They rarely come into contact with ordinary materials. High-energy cosmic neutrinos have also been measured by researchers at the IceCube Neutrino Observatory.
Neutrinos are subatomic particles with no electrical charge and a very low mass. Neutrinos are crucial to physicists because they are numerous, allowing us to use them as a proxy to investigate everything from supernovas (star explosions) to particle evolution.
The Universe is teeming with energetic particles including X rays, gamma rays, and neutrinos. However, the sources of the majority of high-energy cosmic particles remain unknown. An interdisciplinary scientific team has now presented a theory that explains these phenomena: low-activity black holes operate as significant producers of high-energy cosmic particles.
Both gamma rays and neutrinos should be produced by large cosmic-ray accelerators or the Universe’s surrounding surroundings. Their origins, however, remain unknown. Active supermassive black holes (also known as active galactic nuclei), particularly those with large jets, are commonly thought to be the most promising emitters of high-energy gamma rays and neutrinos. Recent analyses, however, have demonstrated that they do not explain the observed gamma rays and neutrinos, implying that alternative source types are required.
The new model demonstrates that non-active, “mellow” black holes, which operate as gamma-ray and neutrino factories, are just as significant as active black holes.
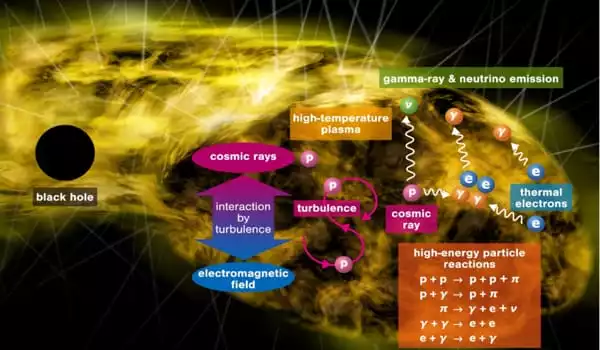
At their cores, all galaxies are believed to have supermassive black holes. A massive amount of gravitational energy is released as stuff falls into a black hole. The gas is heated in this process, resulting in high-temperature plasma. Because of inadequate cooling, the temperature of low-accreting black holes can reach tens of billions of degrees Celsius, and the plasma can generate gamma rays in the megaelectron volt range.
As individual objects, such mellow black holes are dull, yet they are numerous in the Universe. The researchers discovered that the gamma rays produced by low-accreting supermassive black holes may contribute significantly to the reported gamma rays in the megaelectron volt range.
Protons in plasma can be accelerated to energies 10,000 times greater than those attained by the Large Hadron Collider, the world’s largest man-made particle accelerator. Through interactions with matter and radiation, the accelerated protons produce high-energy neutrinos, which can account for the higher-energy component of the cosmic neutrino data. Previous study has shown that this picture can be used to active black holes. A considerable fraction of the observed IceCube neutrinos in a wide energy range can be explained by supermassive black holes, which include both active and inactive galactic nuclei.
The distinction between Hawking’s black hole radiation and thermal radiation released by a black body is that the latter is stochastic in nature, with only its average satisfying Planck’s equation of black-body radiation, whereas the former matches the data better. Thus, thermal radiation retains information about the body that released it, but Hawking radiation appears to carry no such information and is determined solely by the black hole’s mass, angular momentum, and charge (the no-hair theorem). As a result, the black hole information dilemma arises.
Future multi-messenger observational projects will be critical in determining the source of cosmic high-energy particles. The proposed scenario expects gamma-ray analogues to neutrino sources in the megaelectron volt range. Most contemporary gamma-ray detectors are not tuned to detect them; however, future gamma-ray experiments, in collaboration with next-generation neutrino experiments, will be able to detect multi-messenger signals.