An optical cavity is a mirror setup that generates a standing wave cavity resonator for light waves. A laser is the most common type of optical cavity implementation. A mega cavity is something like a laser cavity. It is become more popular to conduct research on microcavities or even nanocavities. They’re also in optical parametric oscillators and some interferometers.
Interferometers are investigative equipment used in a variety of scientific and technical domains. Interferometers are so named because they work by combining two or more sources of light to form an interference pattern that can be monitored and studied; hence the name ‘interfere-o-meter’ or interferometer. Interferometry technology operates on the premise of dividing light into two beams that follow different optical routes and are then combined to produce interference. Interferometric objectives enable the microscope to function as an interferometer; fringes in the sample are visible when it is in focus.
A new notion has been devised that has the potential to help future instruments investigate fundamental science subjects like gravitational waves and dark matter. The concept is presented in an article published in Communications Physics by UK Quantum Technology Hub Sensors and Timing researchers at the University of Birmingham, as well as a related patent application filed by the University of Birmingham Enterprise.
This optical cavity approach offers a route to achieving the enormous laser power needs for future atom-based gravitational wave detectors. By eliminating some of the most severe present technological hurdles, this innovative technique has a real potential to achieve disruptive sensitivity levels in large-scale atom interferometers.
Dr. Rustin Nourshargh
It presents a novel way for enhancing atom interferometers, which are very sensitive devices that utilize light and atoms to achieve ultra-precise measurements. Although difficult to implement, the concept presents a method of overcoming significant technical challenges associated with the pursuit of atom interferometers operating at extreme momentum transfer – a technique that would allow atoms to be placed in a quantum superposition over long distances.
This is critical for providing the sensitivity needed for these devices to explore signals from dark matter and gravitational waves. Exploration of dark matter and detection of gravitational waves from the very early Universe are critical to expanding our collective understanding of fundamental physics.
The new paper, written by Dr. Rustin Nourshargh, Dr. Samuel Lellouch, and colleagues from the School of Physics and Astronomy, describes how synchronization of the input pulses, in order to realize a spatially resolved circulating pulse within the optical cavity, can facilitate a large momentum transfer without requiring drastic increases in available laser power.
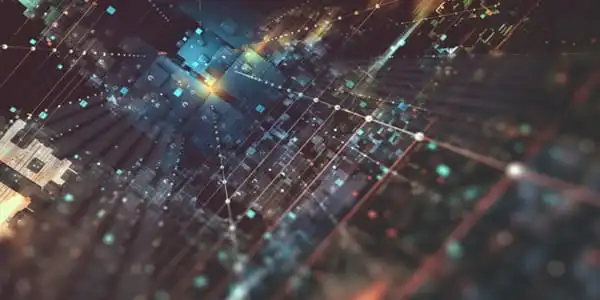
Investigating dark matter and gravitational waves will not only help us comprehend the history of the Universe, but it will also spark fresh ideas for boosting the future sensitivity of atom interferometers. This will also be important for further utilizing atom interferometry in practical applications, such as offering new navigation capabilities by enabling higher resistance against GPS signal loss.
To properly grasp how interferometers work, first, learn more about ‘interference.’ Anyone who has tossed stones into a flat, glassy pond or pool and observed what happens understands interference. When the stones contact the water, they create concentric waves that flow away from the source. And where two or more of those concentric waves overlap, they interfere with each other. This interference can result in a larger, smaller, or no wave at all. The apparent pattern that appears where the waves intersect is just an “interference” pattern. A cutting-edge interferometer can measure distances to within 1 nm (one billionth of a meter, or the width of 10 hydrogen atoms), although it, like any other type of measurement, is vulnerable to inaccuracy.
“This optical cavity approach offers a route to achieving the enormous laser power needs for future atom-based gravitational wave detectors,” stated Dr. Rustin Nourshargh, former doctorate researcher at the University of Birmingham and now Scientist at Oxford Ionics.
“By eliminating some of the most severe present technological hurdles, this innovative technique has a real potential to achieve disruptive sensitivity levels in large-scale atom interferometers,” stated Dr Samuel Lellouch, Research Fellow at the University of Birmingham.