Physicists from the United States and Austria who were looking for evidence of quantum criticality in topological materials discovered one of the most pristine examples yet observed.
In an open access paper published online in Science Advances, researchers from Rice University, Johns Hopkins University, the Vienna University of Technology (TU Wien) and the National Institute of Standards and Technology (NIST) present the first experimental evidence to suggest that quantum criticality – a disordered state in which electrons waver between competing states of order – may give rise to topological phases, “protected” quantum states that are of growing interest for quantum computation.
“The thought that underpins this work is, ‘Why not quantum criticality?'” said study co-author Qimiao Si, a Rice theoretical physicist who has spent two decades researching the interaction between quantum criticality and one of modern physics’ most mysterious processes, high-temperature superconductivity.
“We know quantum criticality provides a setting in which things are fluctuating and from which new states of matter can emerge,” said Si, director of the Rice Center for Quantum Materials (RCQM).
Physicists searching for evidence of quantum criticality in topological materials have found one of the most pristine examples yet observed.
Si and colleagues studied a semimetal made of one part cerium, four parts ruthenium, and six parts tin in the study, which included experimentalist Silke Bühler-Paschen, a longtime collaborator at TU Wien, and Collin Broholm of both NIST and Johns Hopkins. Topological phases have not been observed in CeRu4Sn6, but it is similar to a number of other materials that have. It is also known to be the site of the Kondo effect, which is a strong interaction between the magnetic moments of electrons attached to atoms in a metal and the spins of passing conduction electrons.
Interactions between electrons in typical metals and semiconductors are weak enough that engineers and physicists do not need to consider them when designing a computer chip or other electronic device. This is not the case in “strongly correlated” materials such as Kondo semimetals. The overall behavior of the material – and any device made from it – is based on electron-electron interactions in these. And it is through these interactions that quantum criticality emerges.
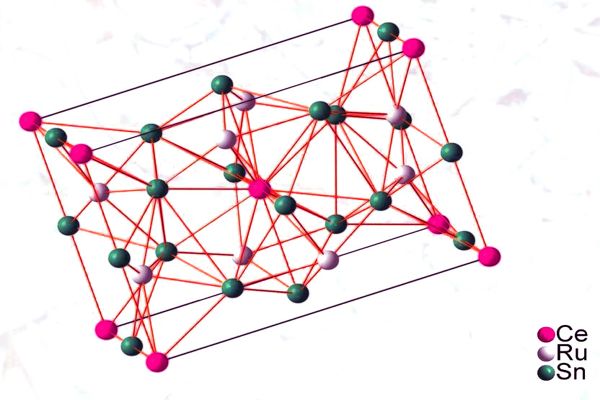
The team used magnetic susceptibility, specific heat, and inelastic neutron scattering measurements to determine the quantum state of CeRu4Sn6 at very low temperatures in experiments at TU Wien and NIST’s Center for Neutron Research. The tests revealed that the material is quantum critical in its native state and does not require fine-tuning.
When strongly correlated materials undergo a phase change at very low temperatures, quantum criticality occurs. At 32 degrees Fahrenheit, the transformation is analogous to freezing liquid phase water into solid phase ice. At critical temperatures, phase changes occur in quantum materials as well, but the phases are quantum in nature. Electrons are ordered one way on one side of the critical point. They are arranged in a different order on the other side. Electrons are fickle at the critical point, constantly fluctuating between competing orders. This is quantum criticality, or the pristine state of CeRu4Sn6.
“Normally, you have to work to achieve that condition,” said Wes Fuhrman, a Johns Hopkins alumnus and one of the study’s lead authors. “Finding these fluctuations is like hitting a bull’s-eye that shrinks in size as the temperature drops. In this case, the semimetal’s dilute electrons appear to act as trail guides to the quantum critical point.”
While CeRu4Sn6 has not been proven to be topological, Si believes it will be in the future, owing to similarities to previous Weyl-Kondo semimetals, a class of materials discovered by Si and Bühler-Paschen in 2017 and further investigated in February.
“To that extent, this work represents the very first step in realizing a still conjectured, conceptual framework in which quantum criticality can be the reason for the emergence of strongly correlated topological semimetals,” Si said.
Quantum states are notoriously fragile; however, in topological materials, patterns of quantum entanglement produce “protected” states that cannot be erased. The immutability of topological states is gaining popularity in quantum computing, which uses quantum states to store and process information. According to Si, the current state of topological materials is similar to that of high-temperature superconductors in the 1990s.
“There are a few materials that have been realized,” said Bühler-Paschen, whose team in Vienna has already discovered the first Weyl-Kondo semimetal material and synthesized the large single crystals needed for the neutron-scattering experiments. “But to really get from a few isolated examples to lots of cases, like we have today for unconventional superconductivity, one needs to have a framework, a foundation.”