Cross-correlation noise spectroscopy was used by physicists to measure minute fluctuations in the electrical current flowing between materials inside silicon solar cells. The researchers discovered critical electrical noise signals that are completely invisible to traditional noise-measurement methods. They were also able to pinpoint the likely physical processes causing the noise, which frequently results in energy loss and decreased efficiency. The technique is a significant new tool for improving material interfaces in order to create a better solar cell.
As society transitions to a renewable energy future, solar panels must convert light into electricity as efficiently as possible. Some cutting-edge solar cells are approaching the theoretical maximum efficiency – and physicists from the University of Utah and the Helmholtz-Zentrum Berlin have discovered a way to improve them even further.
Physicists used a technique known as cross-correlation noise spectroscopy in a new study to measure minute fluctuations in the electrical current flowing between materials inside silicon solar cells. The researchers discovered critical electrical noise signals that are completely invisible to traditional noise-measurement methods. They were also able to pinpoint the likely physical processes causing the noise, which frequently results in energy loss and decreased efficiency.
“Noise measurement on an object is a simple process. You can simply purchase devices that do it. However, the issue that we face is that these devices produce noise”, Kevin Davenport, associate professor of physics at the University of Washington and the paper’s lead author, agreed. “With this cross-correlation technique, we can not only measure the noise of the device but also the noise of our detector and remove it, allowing us to see much, much smaller noise signals.”
The technique, which was published in the journal Scientific Reports, is an important new tool to improve material interfaces for a better solar cell or to analyze inefficiencies in other complicated devices.
Physicists used cross-correlation noise spectroscopy to measure miniscule fluctuations in electrical current flowing between materials inside silicon solar cells. They identified crucial electrical noise signals, completely invisible to conventional noise-measuring methods.
“It’s surprising how important minor efficiency gains are for the industry. Because of the scale of production, even a fraction of a percent improvement translates into billions of dollars “Klaus Lips, professor of physics at Freie Universität Berlin and department head at Helmholtz-Zentrum Berlin, where the solar cells were designed and manufactured, is a co-author.
“In the past, we used the cross-correlation technique to study fairly simple research-grade light-emitting diodes, but the benefits of the method truly came to light in this work,” said Andrey Rogachev, professor of physics at the U and study co-author. “It also extends beyond the solar industry. Each interface between materials in any device with many layers can reduce efficiency in some way. Because it’s so complicated, you have to be extremely discreet in order to explain what’s going on and, more importantly, where the specific noise is occurring. This technique, it turns out, allows us to do just that.”
As is often the case in modern research, a single method was insufficient to comprehend complex devices. Solar cell simulations performed by C.T. Trinh, a postdoctoral researcher at Helmholtz-Zentrum Berlin and co-author of the study, greatly aided in the interpretation of the noise date. Mark Hayward, who was an undergraduate research student at the U and is now a graduate student at the University of California, Irvine, is the final co-author.
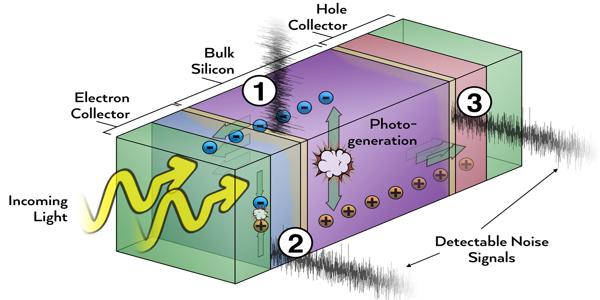
Analyzing noise
The study looked at silicon hetero junction solar cells (HSCs), a high-end type of single-material solar cell that is currently the most efficient on the market, converting 26.7 percent of the light that hits the cell into electricity. In comparison, the cells that make up solar panels on a residential house range in efficiency from 15% to 20%.
Individual particles of light called photons are absorbed by the photoactive layer made of crystalline silicon in an HSC, resulting in pairs of negatively charged electrons and positively charged holes, which are charges caused by missing electrons. An electrical field created by two selective contacts made of hydrogenated amorphous silicon modified with impurities then pulls electrons and holes in opposite directions. This process generates current, which we call electricity.
The problem is that the selective electrode and photo-active silicon do not perfectly match, resulting in defects that trap electrons. To eliminate these flaws in research-grade solar cells like the ones used in the study, the researchers sandwiched an ultrathin layer of pure amorphous silicon between them. Finally, these five layers are sandwiched between two layers of ITO transparent conducting material and gold electrodes.
The efficiency of HSCs is determined by how well the different layers are connected. A minor mismatch between two layers can make it difficult for electrons to get where they need to go, resulting in a noise signal.
“That problem is kind of hidden inside these interfaces, and detecting any kind of signal is extremely difficult. However, the noise technique we employ is extremely sensitive to extremely small individual signals “Davenport stated. He continued, “It’s like listening to a note played by different instruments.” A C-note on a violin and a C-note on a cello are the same note, but they sound different. If you analyze that note, you can glean information about the instrument that produced it, such as the length or material of the strings.
“That’s something we do as well. This broad spectrum of different noise signals and positions along the frequency axis is visible. ‘OK, this part of the note that we see can be attributed to this physical process, and this part is a different physical process,’ we can say” Davenport stated. “However, the device is full of these processes that all generate noise, and it’s extremely difficult to untangle them – it’s like trying to extract a single voice from a 200-person chorus. This technique allows us to remove a large portion of the unwanted signal.”
Mapping inefficiencies
Silicon HSCs are already excellent, but they have limitations. The new technique developed by the research team identified key areas in the device where specific physical processes produce electrical signals. Small changes at these stages could improve the efficiency of these cells and future solar cells in the future. After sorting through the electrical cacophony to find the relevant signals, the physicists ran a simulation to determine what physical processes were occurring at the signal’s location.
Tandem cells are the next generation of solar cells, which are stacks of different photovoltaic materials that are each sensitive to a different part of the sun’s light, allowing such a device to generate more energy. The hot-ticket perovskite material is one proposed device layer. “Together, the new solar cell can exceed the limit of the silicon device by itself, exceeding 30 percent efficiency,” Lips said.
Small losses are important at the cutting edge of efficiency. Material scientists have observed one such loss; the deposition of the transparent ITO modifies the underlying silicon layers, causing defects that reduce the device’s efficiency. One of the major electrical noise signals identified by the researchers in this study occurred at this interface, where charges are trapped and released. Another significant signal occurred as holes passed through a similar barrier on the device’s backside.
“Being able to detect these signals means we can understand their sources and mitigate them,” Davenport explained. The NSF funded the research at the University of Utah, and the DFG funded the research at the Helmholtz-Zentrum.