In a new study, scientists studied magnetic fields in galaxy clusters, revealing the impact of galactic mergers on magnetic-field structures and calling into question earlier assumptions regarding the efficiency of chaotic dynamo processes in the amplification of these fields.
Galaxy clusters are massive, gravitationally bound objects that contain many galaxies, hot gas, and dark matter. They represent some of the largest structures in the cosmos. These clusters can contain hundreds to thousands of galaxies held together by gravity and immersed in enormous halos of hot gas known as the intracluster medium (ICM).
The gravitational attraction of the cluster holds the ICM together, which is primarily made up of ionized hydrogen and helium. Magnetic fields in large-scale formations, like as galaxy clusters, play critical roles in shaping astrophysical phenomena. They have an impact on galaxy formation and evolution, contribute to cosmic ray transmission, play a role in cosmic magnetization, and act as tracers of large-scale structural evolution.
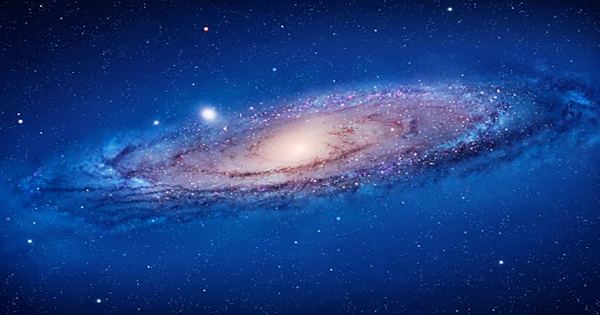
Previous research and simulations have revealed that magnetic fields within clusters develop, showing susceptibility to cluster dynamics and amplification during merging events.
The work, published in Nature Communications, employs a technology known as synchrotron intensity gradient (SIG) to map magnetic fields in clusters, particularly during galaxy mergers. This method offers a unique perspective on magnetic field structures as well as a tool for comparing numerical simulation results to observational data.
Yue Hu, a University of Wisconsin-Madison student, led the investigation. Prof. Alex Lazarian of the University of Wisconsin-Madison, co-author of the study, told Phys.org about his motivation for studying magnetic fields in galaxy clusters, saying, “The focus of my research lies in understanding the role of magnetic fields in astrophysical environments, particularly in magnetized and turbulent media.”
“I’ve spent the last two decades studying magnetic turbulence and reconnection processes with my pupils. The technique for mapping magnetic fields in galaxy clusters is based on theoretical and numerical insights developed during years of research.”
Synchrotron intensity gradient: Synchrotron intensity is the radiation emitted by charged particles, usually electrons, as they spiral along magnetic field lines at relativistic speeds. This phenomenon is referred to as synchrotron radiation.
The SIG approach provides a new perspective by mapping magnetic fields using a process based on the synchrotron intensity gradient. The applicable technology is based on the interaction of magnetic fields and conductive fluids, specifically ionized gas or plasma.
The key concept is that magnetic fields influence the velocity of these fluids, and their resistance to bending makes it easier to determine their orientation. Prof. Lazarian added, “These motions cause velocity gradients and magnetic field variations that are perpendicular to the magnetic field. By detecting these gradients, one may determine the direction of the magnetic field.”
This method of monitoring magnetic fields is innovative and was developed by Prof. Lazarian’s group based on fundamental magnetohydrodynamic studies.
“It utilizes data initially deemed irrelevant for magnetic field studies, allowing us to derive significant results from diverse archival datasets collected for purposes unrelated to magnetic field investigations,” explained Dr. Lazarian.
Mapping Magnetic Fields: The researchers obtained magnetic field maps at the biggest scales ever investigated, focusing on the halos of galaxies within galaxy clusters.
“We proved the accuracy of our technique by comparing the magnetic field directions acquired with our technique to those obtained with the traditional method based on polarization measurement. We also tested the accuracy of SIGs using numerical simulations,” remarked Prof. Lazarian.
The study showed that SIGs provide a novel way to map magnetic fields at unprecedentedly vast scales. The magnetic field structure reveals the complexity of plasma dynamics within merging galaxy clusters.
The discoveries have important implications for our knowledge of cluster dynamics and evolution, providing novel insights into the role of magnetic fields in fundamental processes within galaxy clusters.
Overcoming depolarization: Except for relics, depolarization makes it difficult to map magnetic fields in galaxy cluster regions using typical synchrotron polarization studies. Unlike previous approaches, SIGs are unaffected by depolarization. This study sought to determine whether SIGs and polarization signal the same magnetic field directions when polarization is present.
Yue Hu, the first author, and student, worked with Italian experts Dr. Annalisa Bonafede and Dr. Chiara Stuardi to successfully evaluate magnetic field measurements within relics, proving the trustworthiness of SIG magnetic field maps. Ka Wai Ho, a student of Prof. Lazarian, performed fluid dynamics simulations that confirmed map correctness.
SIGs offer a novel approach to answering long-standing concerns concerning the formation, evolution, and impact of magnetic fields in galaxy clusters without the limitations that standard measurements present.
Heat conduction in ICM: SIGs also enable researchers to test and evaluate existing theories about heat conduction in the ICM and the formation of cooling flows, which is a poorly understood process.
“Heat conduction in ICM’s intracluster plasma (completely ionized gas) is greatly reduced in the magnetic field’s perpendicular direction. Thus, the ability of heat to be transferred in different directions is determined by the structure of the magnetic field. Prof. Lazarian highlighted that changes in heat conductivity influence the production of cold gas streams surrounded by hot gas, known as cooling flows.
Cosmic Ray Acceleration: Cosmic rays are high-energy charged particles that interact intensely with magnetic fields in galaxy cluster halos. Dr. Gianfranco Brunetti, one of the paper’s co-authors, is a top researcher on cosmic ray acceleration processes in galaxy clusters. He is excited about exposing the previously cryptic structure of magnetic fields.
“Clusters of galaxies are known to accelerate cosmic rays through their interaction with moving magnetic fields. The image of this acceleration remains vague and is dependent on magnetic field dynamics,” said Prof. Lazarian.
Furthermore, cosmic rays travel along magnetic field lines, implying that their escape from clusters is impacted by the precise structure of these magnetic fields. The SIG approach can now be used to map the dynamics of magnetic fields within clusters, allowing us to better understand how the universe’s greatest particle accelerators operate.
SIGs, which can scan magnetic fields in locations where polarization information is lost, provide crucial insights into galaxy cluster halos and even bigger synchrotron-emitting objects, such as the recently found Megahalos.
A multinational team, led by Dr. Brunetti of the European Low-Frequency Array (LOFAR), a low-frequency interferometer spanning numerous European countries, recently discovered massive bubbles 30 times the volume of the biggest galactic halo. These structures, known as SIGs, offer the only way to study magnetic fields within these massive cosmic bubbles using LOFAR data. This discovery, according to Italian and Wisconsin experts, is a significant step toward unlocking the cryptic secrets of the universe’s magnetism.
As the astrophysics world awaits the installation of the Square Kilometer Array (SKA) telescope in 2027, the future of magnetic field mapping in galaxy clusters appears bright. The SKA will supply synchrotron intensity for the SIG approach, as well as polarization, which can be used by other methods developed by Prof. Lazarian’s group to analyze the detailed 3D structure of astrophysical magnetic fields.
Prof. Lazarian stated, “The gradient technique is a practical result of a greater understanding of fundamental magnetohydrodynamical processes, prompting us to explore further into these critical processes. While the benefits of fundamental research are not often obvious, improvements in understanding important physical processes cause tectonic changes that effect many areas of science and engineering.”