New research debunks the notion that solid-state qubits must be very dilute in an ultra-clean material to have a long lifetime. Instead, squeeze many rare-earth ions into a crystal, and some will form pairs that function as highly coherent qubits, according to a report published in Nature Physics.
Clean lines and minimalism or old shabby chic? It turns out that the same patterns that dominate the world of interior design are critical when it comes to constructing the building blocks for quantum computers.
One of the most significant challenges to effective quantum computing is the ability to create qubits that maintain their quantum information for long enough to be useful. It is commonly believed that cleanliness is the key to long-lasting qubits, or ‘coherences’. When qubits interact with their environment, they lose quantum information due to a process known as decoherence.
So, according to traditional opinion, keep them away from one another and other potentially harmful influences, and they should live a little longer.
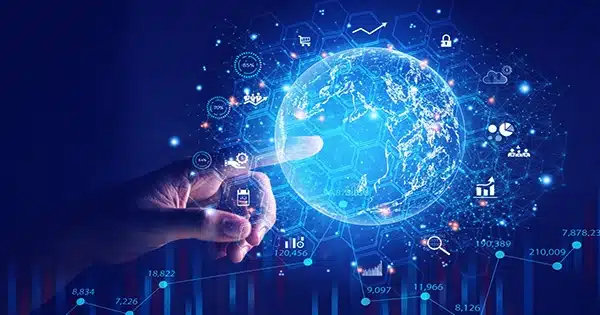
Using a minimalistic approach to qubit design is challenging. Finding suitable ultra-pure materials is not simple. Furthermore, diluting qubits to extremes makes scaling up any resulting technology problematic. Researchers at the Paul Scherrer Institute PSI, ETH Zurich, and EPFL have shown that qubits with long lives can exist in a congested environment.
“In the long term, how to get it onto a chip is a broadly addressed topic for all forms of qubits. Instead of diluting more and more, we’ve demonstrated a novel approach for bringing qubits closer together,” says Gabriel Aeppli, head of PSI’s Photon Science Division and professor at ETH Zürich and EPFL, who led the project.
Picking diamonds from rubbish: The researchers generated solid-state qubits by doping the rare-earth element terbium into yttrium lithium fluoride crystals. They demonstrated that qubit diamonds with substantially longer coherences may be found within a crystal densely packed with rare-earth ions.
“For a given density of qubits, we show that it’s a much more effective strategy to throw in the rare-earth ions and pick the gems from the junk rather than trying to separate the individual ions from each other by dilution,” says Markus Müller, whose theoretical explanations were critical to understanding the puzzling observations.
Qubits, like classical bits, store and process information in 0 or 1 states, with the possibility of superposition. When rare-earth ions are utilized to make qubits, a feature of the individual ions, such as the nuclear spin, which can point up or down, is employed to represent this two-state system.
Pairing up offers protection: The researchers could be successful with a completely different strategy because its qubits are made up of highly interacting pairs of ions rather than single ions. Instead of employing single ions’ nuclear spins, the couples produce qubits by superimposing various electron shell states.
Only a small number of terbium ions form couples within the crystal matrix. “If you throw a lot of terbium into the crystal, by chance, there will be pairs of ions—our qubits. Because these are rather rare, the qubits themselves are quite dilute,” says Adrian Beckert, the study’s lead author.
So, why aren’t these qubits concerned by their untidy surroundings? It turns out that these jewels, due to their physical qualities, are protected from garbage. Because they function at a different characteristic energy, they are unable to exchange energy with single terbium ions, rendering them blind.
“If you make an excitation on a single terbium, it can easily hop over to another terbium, causing decoherence,” Müller said. “However, if the excitation is on a terbium pair, its state is entangled, thus it lives at a different energy and cannot transfer to the single terbium. I’d have to find another pair, but I can’t because the next one is so far away.”
Shining light on qubits: Meanwhile, terbium is a desirable dopant because it is easily stimulated by microwave frequencies utilized in telecommunications. During spin echo testing, a well-established technique for measuring coherence times, the team found unusual peaks corresponding to substantially longer coherences than those on single ions.
“There was something unexpected lurking,” Beckert recalls. With more microwave spectroscopy measurements and rigorous theoretical analysis, they were able to separate these as pair states.
“With the right material, coherence could be even longer.”
As the researchers dug deeper into the nature of these qubits, they were able to comprehend the various ways in which they were protected from their surroundings and sought to improve them. Although the terbium pairs’ excitations may be effectively protected from the action of other terbium ions, nuclear spins on other atoms in the material may still interact with the qubits, causing them to decohere.
To further isolate the qubits from their surroundings, the researchers applied a magnetic field to the material that was calibrated to precisely cancel out the influence of the terbium’s nuclear spin in pairs.
This produced non-magnetic qubit states that were only slightly sensitive to noise from the nuclear spins of nearby ‘junk’ atoms.
Once this level of protection was added, qubit pairs might last up to a hundred times longer than single ions in the same material.
“If we’d set out to look for qubits based on terbium pairs, we wouldn’t have taken a material with so many nuclear spins,” Aeppli said. “What this demonstrates is how effective this technique can be. With the correct material, the coherence may be even greater. The researchers will now use their knowledge of this occurrence to optimize the matrix.