Diamonds are well-known as the hardest substances in existence, a title that has recently been challenged. In principle, altering the arrangement of carbon atoms should result in something even tougher, but no one has yet achieved the pressures required to create a so-called “super diamond”. This could alter, however, since computer modeling indicates the circumstances that may be required.
Diamonds’ strength stems from the way each carbon atom is linked to its four nearest neighbors via covalent bonds, resulting in extraordinarily densely packed atoms. Pure carbon atoms can be arranged in a variety of ways, resulting in solids such as buckyballs and graphene.
The question of whether unusual materials, such as boron nitride, may outperform diamonds remains open, although there is a technique to link carbon atoms that is theoretically stronger than the conventional diamond arrangement. This is known as eight-atom body-centered cubic (BC8) and is expected to resist compression 30% better than conventional diamonds.
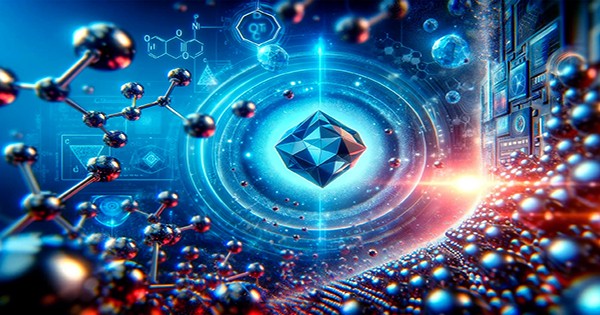
There would undoubtedly be industrial demand for such a material, but it is estimated that pressures of at least 10 million atmospheres (a trillion Pascals) would be required to form the atoms into such a shape. Once created, they should be stable under more normal conditions. Labs have achieved the circumstances that some prior estimations suggested would generate BC8 diamonds, only to discover that these were overly optimistic, leaving scientists wondering how high the pressures would need to be.
If humans cannot create such high circumstances to produce super diamonds, nature is unlikely to have done it either. That is most certainly true on Earth, however, some exoplanets (planets circling other stars) are predicted to be extremely carbon-rich. Pressures at the center of worlds like these may readily meet requirements.
“The extreme conditions prevailing within these carbon-rich exoplanets may give rise to structural forms of carbon such as diamond and BC8,” Professor Ivan Oleynik of the University of South Florida stated. “Therefore, an in-depth understanding of the properties of the BC8 carbon phase becomes critical for the development of accurate interior models of these exoplanets.”
BC8 can also occur in silicon and germanium, the elements just below carbon on the periodic table, and has been synthesized. Using what we know about its formation in these elements, Oleynik and colleagues developed computer models to investigate what would be necessary to make it happen in carbon.
The computations are massive, but using Frontier, the world’s fastest exascale supercomputer, the team believes they have uncovered what is required to make billions of atoms join together correctly. “We predicted that the post-diamond BC8 phase would be experimentally accessible only within a narrow high-pressure, high-temperature region of the carbon phase diagram,” Oleynik stated in a press release.
Pressures of 1,050,000,000,000 Pascals would be necessary at a precise temperature of approximately 6,000 K. Even higher pressures would increase the theoretical temperature range, but not significantly. To get there, the software predicts that regular diamonds will melt into a metastable supercooled carbon liquid, which will then generate BC8s. BC8 crystals, like ice particles in supercooled water, would struggle to form but expand rapidly through nucleation once produced. It remains to be seen whether any apparatus on Earth is capable of achieving this.
If it can, the authors believe BC8 carbon can do more than simply outperform diamonds’ resilience to pressure. “The BC8 structure maintains this perfect tetrahedral nearest-neighbor shape, but without the cleavage planes found in the diamond structure,” stated co-author Dr. Jon Eggert from the Lawrence Livermore National Laboratory. Despite the enormous expenditures of creating something like this, its resilience might be invaluable, as well as provide insights into the internal workings of planets with such cores.