Ultrasound has been used in many medical applications, including imaging, therapy, and drug delivery. More recently, researchers have been exploring its potential in controlling cells and tissues, using a technique called “sonogenetics.” Sonogenetics involves genetically engineering cells to express ion channels that are sensitive to ultrasound waves. When these cells are exposed to ultrasound, the ion channels open or close, which can then trigger specific cellular responses.
A group of researchers has created a method for using ultrasound to selectively manipulate genetically engineered cells. Assume you needed to move a single cell from one location to another. How would you go about it? Perhaps some specialized tweezers? A teeny-tiny shovel?
Individual cell manipulation is, in fact, a difficult task. Some research has been done on so-called optical tweezers, which can move cells around with light beams, but while they are effective at moving a single cell, they are not intended for manipulating larger numbers of cells.
Caltech researchers have developed an alternative: air-filled proteins produced by genetically engineered cells that can be pushed around by ultrasound waves, along with the cells that contain them. A paper describing the work appears in the journal Science Advances.
We’ve previously used these vesicles for imaging, and this time we’ve shown that we can actually use them as actuators to apply force to these objects using ultrasound. What this enables us to do is to move cells around in space using ultrasound in a very selective manner.
Di Wu
The research builds on previous work done in the lab of Mikhail Shapiro, professor of chemical engineering and medical engineering and Howard Hughes Medical Institute investigator.
Shapiro has been using gas vesicles derived from bacteria as an acoustic tag for many years. Some aquatic bacteria use these vesicles, which are air-filled protein capsules, for buoyancy. They also have another useful feature: their air-filled interiors make them visible in ultrasound imagery. Shapiro’s discovery of this property inspired his lab to use gas vesicles as a genetic marker to track the location of individual bacterial cells and to monitor gene-expression activity in mammalian cells deep within the body.
Shapiro and his colleagues have now demonstrated that under the influence of ultrasound, these vesicles can push and pull cells into specific locations. The phenomenon is very similar to how ultrasound in air can be used to suspend and/or move small, light objects. This is because sound waves create pressure zones that act on objects in their vicinity. The physical properties of an object or material determine whether it will be attracted to or repellent to a high-pressure zone. Normal cells are pushed away from areas of higher pressure, but cells containing gas vesicles are drawn to them.
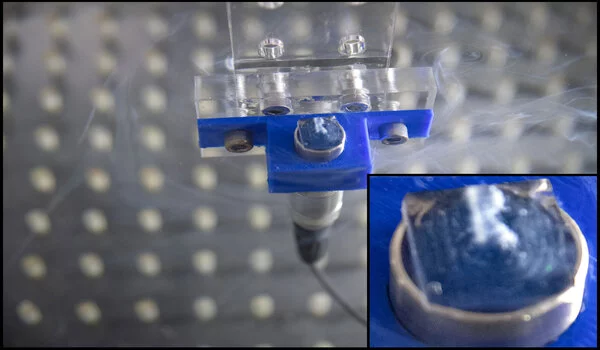
“We’ve previously used these vesicles for imaging, and this time we’ve shown that we can actually use them as actuators to apply force to these objects using ultrasound,” says Di Wu (MS ’16, PhD ’21), the study’s lead author and a research scientist in Shapiro’s lab. “What this enables us to do is to move cells around in space using ultrasound in a very selective manner.”
According to Shapiro and Wu, there are several reasons why you might want to be able to move cells around. For example, tissue engineering – the creation of artificial tissues for research or medical purposes – necessitates the arrangement of specific types of cells in complex patterns. An artificial muscle might need multiple layers of muscle cells, cells that create tendons, and nerve cells, for example.
Another case in which you might want to move cells around is in cell-based therapy, a field of medicine in which cells with desirable properties are introduced into the body. “You’re introducing engineered cells into the body, and they go all over the place to find their target,” Di says. “But with this technology, we potentially have a way to guide them to the desired location into the body.”
As an example, the team demonstrated how cells containing gas vesicles can be forced to clump into a small ball, arranged as thin bands, or pushed to the container’s edges. When the ultrasound pattern was changed, the cells “danced” to take up new positions. They also created an ultrasound pattern that pushed cells into the shape of the letter “R” in a gel and kept them there after it solidified. The resulting image is known as a “acoustic hologram.”
An ultrasound apparatus arranges gas vesicles into the shape of the letter R in solution. Credit: Lance Hayashida/Caltech Wu says one area where their research has the potential for immediate impact is in cell sorting, a process necessary for various kinds of biological and medical research.
“A common way people sort cells now is to engineer them to express a fluorescent protein and then use a fluorescent-activated cell sorter (FACS),” he says. “That is a $300,000 piece of equipment that is bulky, often lives in a biosafety cabinet, and doesn’t sort cells very fast.”
“Acoustic-fluidic sorting, on the other hand, can be accomplished with a tiny little chip that costs around $10. The reason for this distinction is that in fluorescent sorting, you must first measure the gene expression of the cells before moving them. This is accomplished one cell at a time. With gas vesicle expression, the genetics of the cell are directly linked to the force applied to the cell. If they express gas vesicles, they will feel a different force, so we don’t need to check for gas vesicles separately and then move them; we can move them all at once. This greatly simplifies matters.”