Electrical control of quantum phenomena is the use of electric fields or currents to manipulate the quantum states of particles or systems. Quantum computers, which employ quantum mechanics principles, have the potential to revolutionize computing by solving certain problems exponentially faster than classical computers.
A new electrical method for changing the direction of electron flow in some quantum materials could have implications for the development of next-generation electronic devices and quantum computers. Penn State researchers developed and demonstrated the method in materials that exhibit the quantum anomalous Hall (QAH) effect, which is a phenomenon in which electrons flowing along the edge of a material do not lose energy. The team described the work in a paper appearing in the journal Nature Materials.
“As electronic devices get smaller and computational demands get larger, it is increasingly important to find ways to improve the efficiency of information transfer, which includes the control of electron flow,” said Cui-Zu Chang, Henry W. Knerr Early Career Professor and associate professor of physics at Penn State and co-corresponding author of the paper. “The QAH effect is promising because there is no energy loss as electrons flow along the edges of materials.”
Chang was the first to experimentally demonstrate this quantum phenomenon in 2013. This effect is exhibited by QAH insulators, which are a type of topological insulator (a thin layer of film only a few dozen atoms thick) that has been made magnetic so that it only conducts current on its edges. Because the electrons travel in only one direction, the effect is known as dissipationless, which means that no energy is lost in the form of heat.
To make this method effective, we needed to increase the density of the applied current. By narrowing the QAH insulator devices, the current pulse resulted in a very high current density that switched the magnetization direction, as well as the direction of the electron transport route.
Cui-Zu Chang
“In a QAH insulator, electrons on one side of the material travel in one direction, while those on the other side travel in the opposite direction, like a two-lane highway,” Chang said. “Our earlier work demonstrated how to scale up the QAH effect, essentially creating a multilane highway for faster electron transport. In this study, we develop a new electrical method to control the transport direction of the electron highway and provide a way for those electrons to make an immediate U-turn.”
The researchers created a QAH insulator with tailored properties. They discovered that applying a 5-millisecond current pulse to the QAH insulator affects the material’s internal magnetism and causes electrons to change directions. The ability to change direction is critical in quantum technologies for optimizing information transfer, storage, and retrieval. Unlike current electronics, which stores data in a binary state as on or off – as one or zero – quantum data can be stored in a range of possible states at the same time. Changing the electron flow is a critical step in writing and reading these quantum states.
“The previous method to switch the direction of electron flow relied on an external magnet to alter the material’s magnetism, but using magnets in electronic devices is not ideal,” said Chao-Xing Liu, professor of physics at Penn State and co-corresponding author of the paper. “Bulky magnets are not practical for small devices like smartphones, and an electronic switch is typically much faster than a magnetic switch. In this work, we found a convenient electronic method to change the direction of electron flow.”
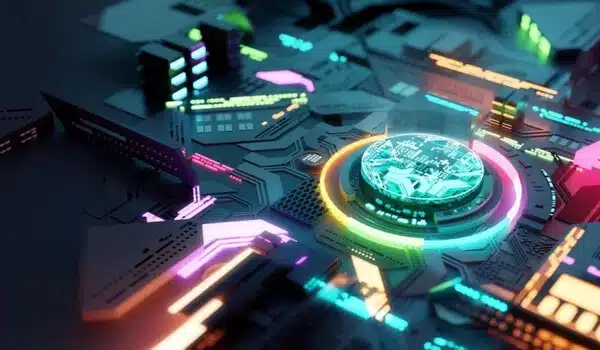
The researchers previously optimized the QAH insulator so that they could take advantage of a physical mechanism in the system to control its internal magnetism.
“To make this method effective, we needed to increase the density of the applied current,” Liu said. “By narrowing the QAH insulator devices, the current pulse resulted in very high current density that switched the magnetization direction, as well as the direction of the electron transport route.”
According to the researchers, the transition from magnetic to electronic control in quantum materials is analogous to the transition that has occurred in traditional memory storage: While older hard drives and floppy disks used magnets to create a magnetic field and write data, newer “flash memory” such as that found in USB drives, solid state hard drives, and smartphones is written electronically. MRAM, a promising new memory scaling technology, is also based on physical mechanisms related to internal magnetism.
The research team also provided a theoretical interpretation of their methodology in addition to the experimental demonstration.
The team is currently investigating how to pause electrons on their path, effectively turning the system on and off. They’re also looking into how to show the QAH effect at higher temperatures.
“This effect, as well as current requirements for quantum computers and superconductors, require very low temperatures near absolute zero,” he said. “Our long-term goal is to replicate the QAH effect at more technologically relevant temperatures.”