Researchers recently discovered a new quantum orbit identification technique that allows photoelectron spectroscopy via tunneling ionization to measure attosecond time decision and subangstrom spatial decision electronic speaker.
Many physical, biological, and chemical processes rely on electron motion in atoms and molecules. Understanding and manipulating these phenomena require an understanding of electron dynamics within atoms and molecules. The traditional method is pump-probe spectroscopy.
A well-known example is the 1999 Nobel Prize in Chemistry, in which femtosecond pumped laser pulses were used to probe the atomic motion involved in chemical reactions. However, because electron motion within atoms and molecules takes attoseconds (10-18 seconds) rather than femtoseconds (10-15 seconds), attosecond pulses are required to probe electron motion. Lasers with pulse durations shorter than 100 attoseconds have become available as a result of the advancement of attosecond technology, allowing for the probing and manipulation of electron dynamics in atoms and molecules.
A new method for identifying quantum orbits enables photoelectron spectroscopy via tunneling ionization to provide attosecond temporal and subangstrom spatial resolution measurement of electron dynamics
Strong-field tunneling ionization is another important method for probing electron dynamics. A strong femtosecond laser is used in this method to induce tunneling ionization, a quantum mechanical phenomenon in which electrons tunnel through the potential barrier and escape from the atom or molecule. This method yields photoelectron-encoded data on ultrafast electron dynamics. Electron dynamics can be observed with attosecond-scale resolution using the relationship between the ionization time and the final momentum of the tunneling ionized photoelectron.
The relationship between ionization-time and tunneling photoelectron final momentum has been theoretically established in terms of a “quantum orbit” model, and its accuracy has been experimentally verified. However, it is unknown which quantum orbits contribute to the photoelectron yield in strong-field tunneling ionization, as well as how different orbits correspond to different momentum and ionization times. Identifying the quantum orbits is therefore critical in the study of ultrafast dynamic processes involving tunneling ionization.
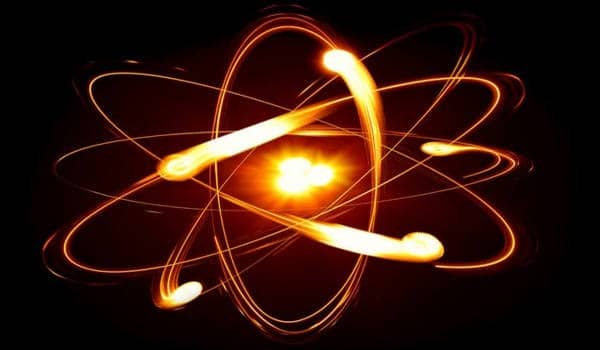
According to Advanced Photonics, Huazhong University of Science and Technology (HUST) researchers proposed a method for identifying and weighing quantum orbits in strong-field tunneling ionization. A second harmonic (SH) frequency is introduced in their scheme to disrupt the tunneling ionization process. Because the perturbation SH is much weaker than the fundamental field, it has no effect on the final momentum of the electron tunneling toward ionization. However, due to the highly nonlinear nature of tunneling ionization, it can significantly alter the photoelectron yield. Because of different ionization times, different quantum orbitals have different responses to the intervening SH field.
The quantum orbits of tunneling ionized electrons can be accurately identified by changing the phase of the SH field relative to the fundamental driving field and monitoring the responses of the photoelectron yield. The mysteries of the so-called “long” and “short” quantum orbits in strong-field tunneling ionization can be resolved using this scheme, and their relative contribution to the photoelectron yield at each momentum can be precisely weighted. This is a significant advancement in the use of strong-field tunneling ionization as a method of photoelectron spectroscopy.
The study, led by HUST graduate students Jia Tan, under the supervision of Professor Yueming Zhou, and Shengliang Xu and Xu Han, under the supervision of Professor Qingbin Zhang, shows that the hologram generated by the multi-orbit contribution from the photoelectronic spectrum can provide valuable information about the tunneled electron’s phase. Its wave packet contains a wealth of information on atomic and molecular electron dynamics.
“Attosecond temporal and subangstrom spatial resolution measurement of electron dynamics is made possible by this new scheme for resolving and weighing quantum orbits,” says Peixiang Lu, HUST professor, vice director of the Wuhan National Laboratory for Optoelectronics, and senior author of the study.