A new theory explains how temperature affects every process. This theory could aid researchers in making accurate predictions in a variety of fields, including biological responses to climate change, infectious disease spread, and food production. Temperature is critical to every biological process. It is true at all scales, from molecules to ecosystems and across all environments, from the very small to the very large.
Until now, there has been no general theory describing how temperature affects life. Researchers led by Jose Ignacio Arroyo, a Santa Fe Institute Postdoctoral Fellow, introduce a simple framework that rigorously predicts how temperature affects living things at all scales in a paper published in the Proceedings of the National Academy of Sciences.
“It’s fundamental,” says SFI External Professor Pablo Marquet, an ecologist at Santiago’s Pontifica Universidad Catolica de Chile. Arroyo’s Ph.D. thesis advisor, Marquet, also contributed to the model. “This can be applied to almost any process that is affected by temperature. We hope it will be a watershed moment.”
I believe that our ability to systematize temperature response has the potential to reveal novel unification in biological processes in order to resolve a variety of controversies.
Professor Chris Kempes
According to Marquet, such a theory could assist researchers in making accurate predictions in a variety of areas, including biological responses to climate change, the spread of infectious diseases, and food production.
Previous attempts to generalize the effects of temperature on biology, according to Marquet, lacked the “big picture” implications built into the new model. The Arrhenius equation, for example, is frequently used by biologists and ecologists to describe how temperature affects the rates of chemical reactions. This method successfully approximates how temperature influences some biological processes, but it falls short of fully accounting for many others, such as metabolism and growth rate.
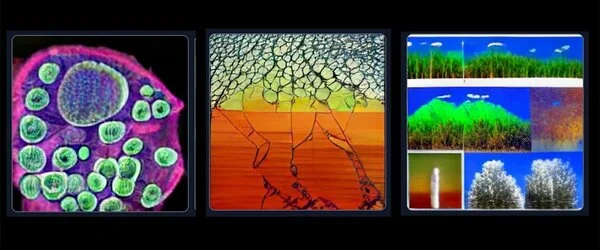
Arroyo initially set out to develop a general mathematical model to predict the behavior of any variable in biology. He quickly realized, however, that temperature was a kind of universal predictor and could guide the development of a new model. He started with a theory in chemistry that describes the kinetics of enzymes, but with a few additions and assumptions, he extended the model from the quantum-molecular level to larger, macroscopic scales.
Importantly, the model combines three elements lacking in earlier attempts. First, like its counterpart in chemistry, it’s derived from first principles. Second, the heart of the model is a single, simple equation with only a few parameters. (Most existing models require a plethora of assumptions and parameters.) Third, “it’s universal in the sense that it can explain patterns and behaviors for any microorganisms or any taxa in any environment,” he says. All temperature responses for different processes, taxa, and scales collapse to the same general functional form.
“I believe that our ability to systematize temperature response has the potential to reveal novel unification in biological processes in order to resolve a variety of controversies,” says SFI Professor Chris Kempes, who, along with SFI Professor Geoffrey West, assisted the team in bridging the quantum-to-classical scales.
The PNAS paper describes predictions from the new model that match empirical observations of a variety of phenomena, including an insect’s metabolic rate, the relative germination of alfalfa, a bacterium’s growth rate, and a fruit fly’s mortality rate.
Arroyo says the group intends to derive new predictions from this model in future publications, many of which were planned for the first publication. “The paper was just getting too big,” he says.