Even though carbon dioxide only makes up 0.0415% of the air we breathe, its atmospheric concentration is about twice what it was before the Industrial Revolution. This gas is excellent at trapping heat and contributes to climate change.
This poses a problem for scientists who are trying to develop artificial trees or other strategies for removing carbon dioxide from the atmosphere directly. A scientific team led by Sandia National Laboratories is working to find a solution to that problem.
The team, led by Sandia chemical engineer Tuan Ho, has been researching how a certain type of clay may absorb and store carbon dioxide using sophisticated computer simulations and laboratory tests.
The researchers released a report describing their preliminary findings in The Journal of Physical Chemistry Letters earlier this week.
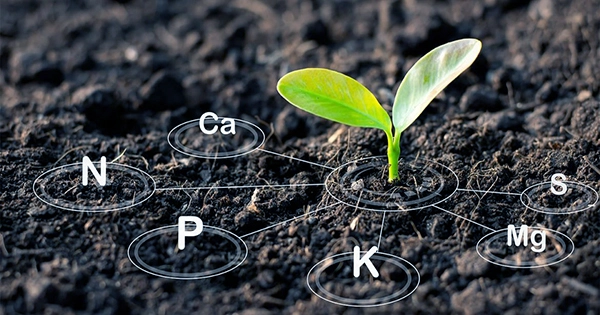
According to Ho, the paper’s principal author, “These fundamental findings offer potential for direct-air capture; that is what we’re aiming toward.” “In nature, clay is quite cheap and plentiful. If this high-risk, high-reward initiative ever results in a solution, it should enable us to drastically lower the cost of direct-air carbon collection.”
Why capture carbon?
In order to lessen the effects of climate change, such as more frequent and severe storms, rising sea levels, and a rise in droughts and wildfires, excess carbon dioxide from the Earth’s atmosphere is captured and stored underground.
This carbon dioxide might be extracted directly from the air, which is more technically difficult, or from fossil fuel-burning power stations, or other industrial facilities, such as cement kilns. One of the least contentious methods being examined for addressing climate change is carbon capture and sequestration.
“We would like low-cost energy, without ruining the environment,” said Susan Rempe, a Sandia bioengineer and senior scientist on the project. “We can reduce the amount of carbon dioxide we produce by how we live, but we have no control over what our neighbors do. Direct-air carbon capture is crucial for lowering atmospheric carbon dioxide levels and reducing the carbon dioxide that our neighbors emit.”
Ho suggests that carbon dioxide may be captured using clay-based devices similar to sponges, and then the captured carbon dioxide could be “squeezed” out of the sponge and injected underground. Or the clay may be utilized more as a filter to sequester carbon dioxide from the air.
Clay is not only affordable and abundantly available, but it also has a high surface area, is stable, and is made up of numerous minuscule particles with fissures and crevasses that are roughly a hundred thousand times smaller in diameter than human hair. According to Rempe, these minuscule spaces are known as nanopores, and they are capable of changing their chemical properties.
Rempe has previously researched carbon dioxide collecting nanostructured materials. She was actually a member of the group that investigated a biological catalyst for converting carbon dioxide into water-stable bicarbonate, designed a thin, nanostructured membrane to shield the biological catalyst, and won a patent for their bio-inspired, carbon-catching membrane. Naturally, this membrane is not created from cheap clay and was first intended to be used in industrial settings or fossil fuel-burning power plants, according to Rempe.
“These are two complementary possible solutions to the same problem,” she said.
How to simulate the nanoscale?
A type of computer simulation called molecular dynamics examines the motions and interactions of atoms and molecules at the nanoscale. Scientists can determine a molecule’s stability in a specific environment, such as water-filled clay nanopores, by looking at these interactions.
Ho asserted that “molecular simulation” is a potent tool for researching interactions at the molecular level. The purpose of using this knowledge to develop a clay material for carbon-capture applications is to completely understand what is happening between carbon dioxide, water, and clay.
According to Ho’s molecular dynamics simulations, carbon dioxide can be significantly more stable in wet clay nanopores than in plain water in this situation. This is due to the fact that water atoms do not equally distribute their electrons, leaving one end slightly positively and the other slightly negatively charged. However, Rempe noted that carbon dioxide atoms do share electrons equally and that the gas is more stable when it is close to other comparable molecules, such as the silicon-oxygen regions of clay.
Ho noted that research done lately by associates from Purdue University under the direction of Professor Cliff Johnston demonstrated that water contained in clay nanopores has a greater capacity to absorb carbon dioxide than free water.
Nabankur Dasgupta, a postdoctoral researcher at Sandia, also discovered that the reaction between carbon dioxide and carbonic acid is more favorable and requires less energy inside the oil-like regions of nanopores than it does in plain water, according to Ho. Ultimately, the oil-like sections of clay nanopores make it possible to capture more carbon dioxide and store it more easily by making this conversion favorable and requiring less energy, he added.
This suggests that clay is a good substance for absorbing carbon dioxide and changing it into another molecule, according to Rempe. “And because we know why, synthesis experts and engineers can change the material to improve that. The tests that test new hypotheses can also be guided by the simulations.”
He noted that the project’s further phases will involve using molecular dynamics models and experiments to ascertain how to get carbon dioxide back out of the nanopore. They intend to conceptualize a clay-based direct-air carbon capture system by the end of the three-year research.