A study of the energy-making process in plants could aid in the development of crops that are more resistant to stress or bacteria that produce pharmaceuticals. A team of scientists led by Imperial College London and the Queen Mary University of London has mapped a critical process in plant energy conversion.
The findings, which were published today in Nature Communications, could help scientists improve the resilience of important crops and engineer bacteria that can produce useful compounds such as pharmaceuticals more sustainably.
All cells, plant and animal alike, use adenosine triphosphate (ATP) as their energy currency. Animals, such as humans, produce ATP through respiration, whereas plants use photosynthesis to convert sunlight into ATP.
A research team developed a computer model to better understand how plants make adjustments to store energy produced by photosynthesis. Understanding these changes could aid in the development of new plants that can withstand rising temperatures caused by climate change.
A study into the energy-making process in plants could help engineer crops more resistant to stress or bacteria that produce pharmaceuticals.
All cells, plant and animal alike, use adenosine triphosphate (ATP) as their energy currency. Animals, such as humans, produce ATP through respiration, whereas plants use photosynthesis to convert sunlight into ATP.
A research team developed a computer model to better understand how plants make adjustments to store energy produced by photosynthesis. Understanding these changes could aid in the development of new plants that can withstand rising temperatures caused by climate change.
With lab experiments, the team confirmed the mathematical model’s accuracy. Their findings were recently published in Nature Plants. “We added an important piece to the puzzle of plant metabolism,” said Helmut Kirchhoff, a professor in WSU’s Institute of Biological Chemistry and the team’s leader. “If we incorporate our model into the larger picture, it could provide a good path for how to improve plants for specific environments.”
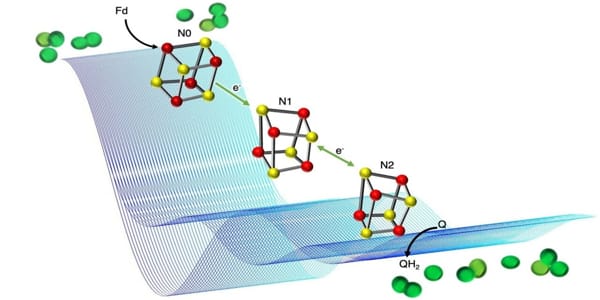
Mapping electron transfer
PS-C1 is known to be used by plants, including many important crop plants, to increase ATP production during times of high stress, allowing them to continue growing in difficult conditions such as low light, drought, or high temperatures.
The researchers behind the new study have now mapped how an electron transfer process required for establishing a proton gradient in PS-C1 works, revealing important details about how plants and photosynthetic bacteria can gain extra energy.
“With this study, we contribute to understanding how cells convert the potential energy in proton gradients into chemical energy—a process that underpins nearly all life on Earth,” said lead researcher Dr. Maxie Roessler of Imperial’s Department of Chemistry.
Dr. Guy Hanke of Queen Mary University, who is also a co-lead, added: “Finally, we hope that understanding PS-C1 function will allow us and other researchers to manipulate photosynthetic bacteria for biotechnological purposes. This could include helping to meet the energy demands required to produce high-value compounds like pharmaceuticals in a more sustainable manner.”
The research team hopes that a better understanding of PS-C1 will eventually aid in the development of crops that can withstand the stresses caused by climate change, such as temperature fluctuations and drought.
Harnessing extra energy resources
PS-C1 is embedded in plant chloroplast membranes, allowing it to control the movement of molecules across the membrane. PS-C1 must act as a “proton pump” to create the proton gradient across the membrane, but how it does so has been difficult to study due to PS-large C1’s and complex molecular machines.
To investigate PS-C1, the researchers used an electron paramagnetic resonance (EPR) spectroscopy technique to map the position of single (unpaired) electrons. The movement of protons is powered by the transfer of electrons between parts of PS-C1, so mapping the electron transfer path can help provide insight into how protons move across the membrane.
The researchers hope that this fundamental study will lead to a better understanding of how this molecular machine works and potential ways to harness its ability to generate extra energy resources for photosynthesis using plants and bacteria. Katherine Richardson et al. published “Functional basis of electron transport within photosynthetic complex I” in Nature Communications.