Engineers at Duke University have created a scalable soft surface that can constantly reshape itself to mimic natural objects. The surface can learn to adapt to obstacles such as broken elements, unexpected constraints, or changing environments by relying on electromagnetic actuation, mechanical modeling, and machine learning to form new configurations.
The study is published in the journal Nature.
“We’re motivated by the idea of controlling material properties or mechanical behaviors of an engineered object on the fly, which could be useful for applications such as soft robotics, augmented reality, biomimetic materials, and subject-specific wearables,” said Xiaoyue Ni, assistant professor of mechanical engineering and materials science at Duke. “We’re focusing on engineering the shape of matter that hasn’t been predetermined, which is a difficult task, especially for soft materials.”
Previous work on morphing matter, according to Ni, hasn’t typically been programmable; it’s been programmed instead. That is, soft surfaces equipped with designed active elements can shift their shapes between few shapes, like a piece of origami, in response to light or heat or other stimuli triggers. In contrast, Ni and her laboratory wanted to create something much more controllable that could morph and reconfigure as often as it likes into any physically possible shapes.
This is the first artificial soft surface that is fast enough to accurately mimic a continuous shape-shifting process in nature. One key advance is the structural design that enables an unusual linear relationship between the electrical inputs and the resulting shape, which makes it easy to figure out how to apply voltages to achieve a wide variety of target shapes.
Xiaoyue Ni
The researchers began by laying out a grid of snake-like beams made of a thin layer of gold encapsulated by a thin polymer layer to create such a surface. Individual beams are only eight micrometers thick – roughly the thickness of a cotton fiber – and less than one millimeter wide. Because the beams are light, magnetic forces can easily and quickly deform them.
The surface is placed in a low-level static magnetic field to generate local forces. Voltage changes generate a complex but easily predictable electrical current along the golden grid, which drives the grid’s out-of-plane displacement.
“This is the first artificial soft surface that is fast enough to accurately mimic a continuous shape-shifting process in nature,” Ni said. “One key advance is the structural design that enables an unusual linear relationship between the electrical inputs and the resulting shape, which makes it easy to figure out how to apply voltages to achieve a wide variety of target shapes.”
The new “metasurface” demonstrates a wide range of morphing and mimicking abilities. It generates bulges that rise and move around the surface like a cat trying to get out from under a blanket, oscillating wave patterns, and an accurate representation of a liquid drop dripping and plopping onto a solid surface. And it can generate these shapes and behaviors at any speed or acceleration, so it can reimagine that trapped cat or dripped droplet in slow motion or fast forward.
The contortionist surface can learn to recreate shapes and patterns on its own with cameras monitoring the morphing surface. By slowly adjusting the applied voltages, a learning algorithm takes in 3D imaging feedback and figures out what effects the different inputs have on the metasurface’s shape.
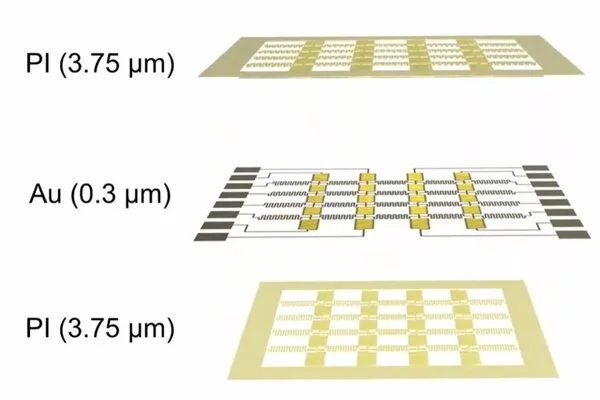
In the paper, a human palm spotted with 16 black dots slowly shifts under a camera, and the surface mirrors the movements perfectly.
“The control doesn’t need to understand the physics of the materials; it just takes small steps and watches to see if it’s getting closer to the target or not,” Ni explained. “It currently takes about two minutes to achieve a new shape, but we hope to improve the feedback system and learning algorithm to the point where it’s nearly real-time in the future.”
Because the surface learns by trial and error, it can adapt to damage, unexpected physical constraints, or environmental change. In one experiment, despite having one of its beams cut, it quickly learns to mimic a bulging mound. In another, despite a weight attached to one of the grid’s nodes, it manages to mimic a similar shape.
There are numerous immediate opportunities to expand the soft surface’s scale and configuration. An array of surfaces, for example, can scale up to the size of a touching screen. Alternatively, more precise fabrication techniques can reduce the size to one millimeter, making it more suitable for biomedical applications.
Ni’s long-term goal is to develop robotic metasurfaces with integrated shape-sensing functions capable of performing real-time shape mimicking of complex, dynamic surfaces found in nature, such as water ripples, fish fins, and the human face. The lab may also investigate the possibility of incorporating additional components into the prototype, such as on-board power sources, sensors, computational resources, or wireless communication capabilities.
“In addition to pursuing programmable and robotic materials, we envision future materials will be able to alter themselves to serve functions dynamically and interactively,” Ni said. “Such materials, like the microbots in Big Hero 6, can sense and perceive user requirements or information, and then transform and adapt to meet the real-time needs of their specific performance. The soft surface could be used as a teleoperated robot, dynamic 3D display, camouflage, exoskeleton, or other smart, functional surfaces capable of working in harsh, unpredictable environments.”