In the ever-changing landscape of condensed matter physics, a recent breakthrough has emerged from the joint efforts of researchers at the Peter Grünberg Institute (PGI-1), École Polytechnique Fédérale de Lausanne, Paul Scherrer Institut in the country of Switzerland, and the Jülich Centre for Neutron Science.
This collaborative effort, directed by Stefan Blügel, Thomas Brückel, and Samir Lounis, has delved into hitherto unknown magnonic characteristics within Mn5Ge3, a three-dimensional ferromagnetic material.
Topology, a key idea in contemporary physics, has already transformed our understanding of electrons in solids. Topology has a broad impact, ranging from quantum Hall effects to topological insulators. In this environment, the emphasis has switched to magnons—collective precession of magnetic moments—as potential bearers of topological phenomena. Magnons, being bosons, can display unique properties similar to their fermionic counterparts.
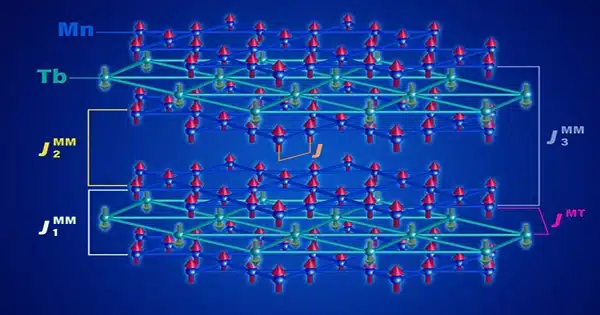
The researchers wanted to investigate the magnetic characteristics of Mn5Ge3, a 3D centrosymmetric ferromagnet. They discovered the material’s unique magnon band structure using density functional theory calculations, spin model simulations, and neutron scattering tests.
The central discovery was the occurrence of Dirac magnons with an energy gap, which is related to Dzyaloshinskii-Moriya interactions. This interaction, as identified inside the material, is responsible for causing a gap in the magnon spectrum.
Mn5Ge3 is a three-dimensional material with gapped Dirac magnons because the gap may be adjusted by rotating the magnetization direction in response to an applied magnetic field. This gap, both theoretically and empirically proved, highlighted the topological nature of Mn5Ge3 magnons.
The research team’s discoveries not only advance our understanding of topological magnons but also highlight Mn5Ge3 as a possible game changer in the field of magnetic materials.
The complicated interaction of components discovered in Mn5Ge3 gives up new possibilities for developing materials with specific magnetic characteristics. Because the material’s magnetic characteristics can be finely adjusted, the idea of incorporating these topological magnons into novel device concepts for practical applications becomes more appealing.
As the scientific community continues to push the boundaries of condensed matter physics, this work represents a critical step toward deciphering the secrets of magnetic materials. The study’s implications not only broaden our understanding of magnons but also pave the path for future technologies that will benefit from their unique quantum features.