Quantum computing is a promising field of research with the potential to revolutionize the way we solve complex problems that are currently beyond the capabilities of classical computers. However, one of the major challenges in building practical quantum computers is the issue of quantum decoherence, which occurs when the fragile quantum states that encode information interact with their environment and lose their coherence.
According to an international team of researchers, a new type of heterostructure of layered two-dimensional (2D) materials may enable quantum computing to overcome key barriers to widespread application.
The researchers were led by a team from the Penn State Center for Nanoscale Science (CNS), one of 19 Materials Research Science and Engineering Centers (MRSEC) funded by the National Science Foundation in the United States. Their findings were published in Nature Materials.
A typical computer is made up of billions of transistors, known as bits, which are controlled by binary code (“0” = off and “1” = on). Based on quantum mechanics, a quantum bit, also known as a qubit, can be both a “0” and a “1” at the same time. This is known as superposition, and it allows quantum computers to be more powerful than traditional computers. However, there is a problem with building a quantum computer.
Quantum computing is a very hot topic right now, and people are thinking about how to build a quantum computer with less error in computation. A topological quantum computer is one appealing method. However, developing the right materials for topological quantum computing is critical.
Jun Zhu
“IBM, Google, and others are attempting to build and scale up quantum computers based on superconducting qubits,” said Jun Zhu, a Penn State professor of physics and the study’s corresponding author. “A key problem in quantum computing is minimizing the negative effect of a classical environment, which causes error in the operation of a quantum computer.”
A solution to this problem may be found in a topological qubit, an exotic version of a qubit. “Topological superconductor-based qubits are expected to be protected by the topological aspect of superconductivity and thus more robust against the destructive effects of the environment,” Zhu explained.
A topological qubit is related to topology in mathematics, where a structure undergoes physical changes such as being bent or stretched while retaining its original properties. It is a theoretical type of qubit that has not yet been realized, but the basic idea is that certain materials’ topological properties can protect the quantum state from being disturbed by the classical environment.
According to Cequn Li, a graduate student in physics and the study’s first author, topological quantum computing is currently receiving a lot of attention.
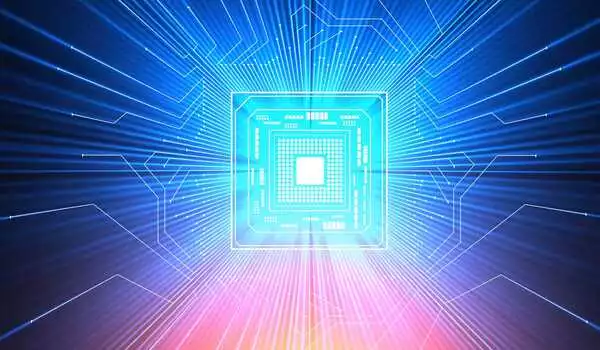
“Quantum computing is a very hot topic right now, and people are thinking about how to build a quantum computer with less error in computation,” Li explained. “A topological quantum computer is one appealing method. However, developing the right materials for topological quantum computing is critical.”
The researchers in the study took a step in this direction by developing a type of layered material known as a heterostructure. The study’s heterostructure consists of a layer of a topological insulator material, bismuth antimony telluride or (Bi,Sb)2Te3, and a layer of a superconducting material, gallium.
“We devised a novel measurement technique to investigate proximity-induced superconductivity at the surface of the (Bi,Sb)2Te3 film,” Zhu explained. “A key mechanism for realizing a topological superconductor is proximity-induced superconductivity. Our findings show that it does occur on the surface of the (Bi,Sb)2Te3 film. This is the first step toward creating a topological superconductor.”
However, creating such a topological insulator/superconductor heterostructure is difficult. “It’s usually difficult because different materials have different lattice structures,” Li explained. “Also, when two materials are combined, they may chemically react with one another, resulting in a messy interface.”
Therefore, the researchers are using a synthesis technique known as confinement heteroepitaxy, which is being explored at MRSEC. This involves inserting a layer of epitaxial graphene, which is a sheet of carbon atoms of one or two atoms thick, between the gallium layer and the (Bi, Sb)2Te3 layer. Li notes this enables the layers to interface and combine, like snapping Lego blocks together.
“Graphene acts as a chemical barrier between these two materials,” Li explained. “As a result, there is no reaction between them, and we have a very nice interface.” Furthermore, the researchers demonstrated that this technique is scalable at the wafer level, making it an appealing option for future quantum computing. A wafer is a round slice of semiconductor material that serves as a microelectronics substrate.
“Our heterostructure has all of the elements of a topological superconductor, but perhaps more importantly, it is thin film and potentially scalable,” Li explained. “As a result, a wafer scale thin film has enormous potential for future applications, such as the construction of a topological quantum computer.”
This research was a combined effort of the CNS’s IRG1 – 2D Polar Metals and Heterostructures team, led by Zhu and Joshua Robinson, professor of materials science and engineering at Penn State. Other faculty involved in the research include Cui-Zu Chang, Henry W. Knerr Early Career Professor and associate professor of physics, and Danielle Reifsnyder Hickey, assistant professor of chemistry and materials science and engineering.
“This was remarkable teamwork by our MRSEC’s IRG1 team,” Zhu said. “The Robinson group used confinement heteroepitaxy to grow the two atomic layer gallium film, the Chang group used molecular beam epitaxy to grow the topological insulator film, and the Reifsnyder Hickey group and Materials Research Institute staff performed atomic scale characterization of the heterostructure and devices.”
The next step is to perfect the process and take another step closer to realizing a topological quantum computer.
“The material is critical, so our collaborators are working to improve it,” Li explained. “This results in greater consistency and higher quality. And our group is attempting to create more advanced devices on these types of heterostructures in order to investigate the signatures of topological superconductivity.”