Diamonds ride rockets to the Earth’s surface after being forged at high temperatures and pressures more than 150 kilometers below in the mantle: thin channels of magma called kimberlite that can erupt at the speed of sound. Surprisingly, most kimberlite pipelines are discovered in the remote, ancient innards of continents. They are located far from where most other eruptions occur: at the boundaries of tectonic plates and near mantle plumes, which generate volcanic hot spots like Hawaii or Yellowstone. “How on Earth did these get here?” Thomas Gernon, a geologist at the University of Southampton, wonders. “There was an elephant in the room that no one could explain.”
Gernon and his colleagues now believe they have. They believe the timing and location of these diamond-bearing eruptions indicate they are the result of supercontinent breakup, which creates swirling turbulence in the viscous mantle rock below. According to the researchers, these swells ripple beneath the continents like slow-motion tidal waves of rock, traversing hundreds of kilometers over millions of years and occasionally sparking kimberlite eruptions. “Kimberlites appear to be responding to supercontinent rhythms,” Gernon explains.
The discovery, which was published today in Nature, is about more than diamonds and kimberlites, according to Folarin Kolawole, a structural geologist at Columbia University who was not involved in the study. It implies that tectonic activity near the Earth’s surface can influence mantle behavior on a larger scale than previously assumed. It also suggests that underground waves maintain the borders of newly divided continents volcanically active for far longer than predicted, which could explain other volcanic rocks previously attributed to mantle plumes. “This gives us a way forward—a hypothesis to test,” says Kolawole.
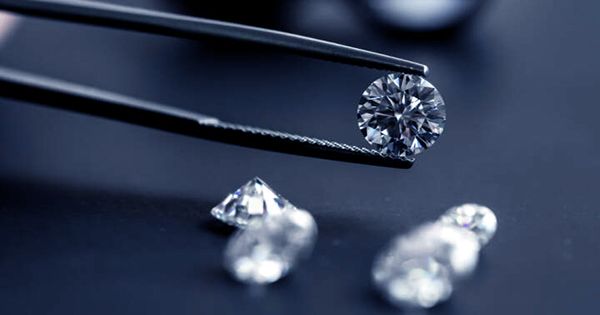
Kimberlite, named after a mine in Kimberley, South Africa, which generated the majority of the world’s diamonds in the late 1800s, is formed when mantle rocks melt into dense magma rich in magnesium, water, and carbon dioxide. CO2 and water combine to form gas bubbles that propel a small plug of magma to the surface, much like a cork popping off a bottle of champagne.
The pipelines are only a few meters across at depth, but when they burst, they inflate into carrot-shaped cones, leaving craters hundreds of meters across. “It’s going to be quite catastrophic when it reaches the Earth’s surface,” says Emma Humphreys-Williams, a geochemist at London’s Natural History Museum. Along the journey, the magma picks up hitchhikers from the upper mantle and continental crust, including diamonds, which are rich in information about the deep Earth’s activities. “There’s a whole element of Earth history that would be lost if we didn’t have kimberlites,” Kelly Russell of the University of British Columbia explains.
Although there are thousands of documented kimberlite pipelines on Earth, none have erupted in recorded human history. The great majority have been around for hundreds of millions of years. “All of us volcanologists would be willing to chip in and have a new eruption of kimberlites,” Russell says.
Gernon found himself returning to the topic of why these eruptions only occur in cratons—the old, frigid interiors of continents—during COVID-19 lockdowns. Although some studies suspected mantle plumes were the cause, numerous kimberlite occurrences do not correspond to recognized hot spot tracks. Furthermore, geochemical examination of isotopes in mantle plume rocks indicates that they originated in the lower mantle, but current kimberlite analyses indicate a shallower origin.
Gernon and his co-authors noted that the timing of kimberlite eruptions seemed to coincide with major plate tectonic events. They reconstructed continental plate movement over the last 500 million years by comparing the rate of continental rifting to kimberlite production bursts. Kimberlite eruptions appeared to peak 26 million years after a continental fracture, according to the data. “That was both curious and jaw-dropping,” Gernon says.
They then focused on the geologic history of kimberlite deposits in southern Africa and South America that formed after the breakup of the Gondwana supercontinent 120 million years ago, as well as those in North America that formed after Pangaea’s earlier breakup. The kimberlite volcanoes grew farther away from the rift throughout time, with the clusters migrating about 20 kilometers every million years.
Gernon and his colleagues believe they understand what motivates this movement. As continents separate, heated mantle rocks rise to fill the void. However, as they collide with the cold continental edges of the gap, they cool and sink, forming whirling convective patterns. According to computer modeling, these vortices flow along the keels of the continents, removing their mushy roots and forming a rock mix suitable for melting into kimberlite. The models show the waves crawling along at around one-millionth of a snail’s pace, which corresponds to the propagation of kimberlites. “It’s a really clever idea,” says geodynamicist Jeroen van Hunen of Durham University. “It all makes perfect sense.”
It’s also a significant assertion, because the enormous waves would tear 40 kilometers of rock from the continents’ bases. However, other data appears to support it. Kimberlites, for example, not only move out of rifts over time, but their isotope mix alters as well, from patterns that mimic a mantle-crust mix where the wave first breaks to a more uniform upper mantle composition as the wave dies out. And, in the southern African Kaapvaal craton, for example, the continent experienced several kilometers of uplift around the same time as the kimberlite eruptions. The uplift indicates that the wave was underfoot at the time, removing the continent’s undercarriage and allowing it to rise like a hot air balloon losing its weight. “This evidence is really compelling when taken together,” Gernon says.
Given how noisy the data is and how much Earth’s peculiarities change from place to place, Karen Smit, a geochemist at the University of the Witwatersrand, believes the team is unlikely to have discovered a single explanation for kimberlites. “It’s a reasonable model.” I’m just not sure if that association exists on a global scale.” However, Kolawole believes the findings may spur additional research in areas such as the Gulf of California and the Red Sea, where incipient rifting may be causing the deep waves revealed by seismic measurements. He adds that the hypothesis could also explain other volcanic deposits traditionally attributed to plumes.
According to Gernon, commercial diamond miners may be most interested in the study. According to him, it could assist predict the location of unknown kimberlites. “You should be able to pinpoint, roughly, the sweet spot for diamonds.”