Earthquakes are notoriously difficult to predict, as are the usually less-severe aftershocks that frequently follow a major seismic event. Greg McLaskey ’05, associate professor of civil and environmental engineering at Cornell Engineering, and members of his research group model earthquakes in the Bovay Laboratory Complex and have developed a method for simulating the type of delayed seismic activity that occurs after an earthquake.
McLaskey’s team studied delayed earthquake triggering using a hybrid sample made up of plastic blocks and powdered rock in order to better understand the mechanisms of aftershocks and, ultimately, the forces that precede a major earthquake.
McLaskey is senior author of “Creep Fronts and Complexity in Laboratory Earthquake Sequences Illuminate Delayed Earthquake Triggering,” published in Nature Communications. Co-lead authors are Sara Beth Cebry, M.Eng. ’18, a doctoral student in civil and environmental engineering; and Chun-Yu Ke, Ph.D. ’21, now a postdoctoral researcher at Pennsylvania State University.
Even in the lab, it’s difficult to study because they happen so quickly. The earthquake is essentially a rupture, a crack that propagates through the Earth at 3 kilometers (1.8 miles) per second.
McLaskey
Aftershocks can occur anywhere from a few hours to several years after a major seismic event and are caused by complex and poorly understood triggering mechanisms. Because these events occur miles below the surface, direct measurement of the shifting rock is rarely possible.
“Even in the lab, it’s difficult to study because they happen so quickly,” McLaskey said. “The earthquake is essentially a rupture, a crack that propagates through the Earth at 3 kilometers (1.8 miles) per second.”
McLaskey and his colleagues have modeled seismic activity on a relatively large scale, such as pressing two 3-meter-long slabs of granite together with more than 2 million pounds of force. However, for this project, the model was significantly scaled down.
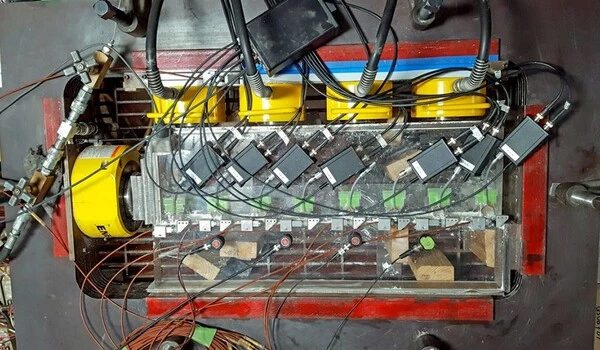
The team used plastic blocks approximately 2 ½ feet in length, with quartz dust in between the blocks to simulate the type of friction occurring between gargantuan tectonic plates 15 kilometers below the Earth’s surface.
“Quartz powder is essentially ground-up sand,” McLaskey explained. “If you had granite grinding against itself, you’d get something like that, so we think it’s pretty representative.”
Cebry and Ke tested a variety of materials to find the right combination of plastic and rock dust to produce aftershock-like activity, albeit on a much smaller scale. Cebry described their formula as having “just the right amount of interest” to be useful.
“The cool thing is, we were able to get one part of the sample to slip rapidly, emit seismic waves, cause a small earthquake, and then there’d be a delay,” McLaskey explained. “The other end of the sample would then rupture. It was similar to an aftershock.”
“Creep fronts” – alluded to in the title of the paper – are the key, McLaskey said. These are sections of faults that slip super slowly and at different rates along the fault, but result in significant changes in the subsurface.
“In California, for example, there are a lot of faults where you see that maybe the sidewalk, after 10 years, has shifted a little bit, but there was no earthquake,” he said. “It was just creeping along.”
Instead of the subsurface rock sliding at a constant velocity, one part of the fault slips faster than another, and the intersection between the faster and slower slipping sections moves, according to McLaskey. “The behavior of the rock on one side is migrating over to affect the behavior on the other side,” he explained.
Based on their modeling, the group discovered that the speed and strength of creep fronts are sensitive to fault stress levels from previous earthquakes. These could be measured and used as local stress meters to help predict seismic events in the future.
“Predicting earthquakes is still a long way off,” McLaskey said, “but I think we have a better understanding of one key aspect of the physics with this work.”