Solid-state batteries are one technology that is generating a lot of interest when it comes to the future of energy. A solid-state battery has a higher energy density than a liquid electrolyte solution-based Li-ion battery. Because there is no danger of explosion or fire, there is no need for safety components, which saves space. Then we have more room to put more active materials in the battery, which increases battery capacity.
Because only a few batteries are required, a solid-state battery can increase energy density per unit area. As a result, a solid-state battery is ideal for creating a high-capacity EV battery system of module and pack.
Solid-state batteries pack a lot of energy into a small space, but their electrodes aren’t very good at staying connected to their electrolytes. Liquid electrolytes can reach every nook and cranny of an electrode to spark energy, but they take up space without storing energy and eventually fail. At the atomic level, researchers are now putting solid electrolytes in contact with electrodes made of strategically arranged materials, and the results are helping to drive better solid-state battery technologies.
Researchers are now putting solid electrolytes in touch with electrodes made of strategically arranged materials – at the atomic level – and the results are helping drive better solid-state battery technologies.
Controlling the atomic alignment of solid materials can improve the cathode-solid electrolyte interface and stability in solid-state batteries, according to a new study led by University of Illinois Urbana-Champaign materials science and engineering professor Paul Braun, postdoctoral research associate Beniamin Zahiri, and Xerion Advanced Battery Corp. director of research and development John Cook. The findings have been published in the journal Nature Materials.
“With batteries, it’s not just the materials that matter, but also how the atoms on those materials’ surfaces are arranged,” Zahiri explained. “Solid-state battery electrodes currently use materials with a wide range of surface atom arrangements. As a result, there appears to be an infinite number of electrode-solid electrolyte contact interface possibilities, each with a different level of chemical reactivity. We want to know which configurations result in practical improvements in battery cycle life, energy density, and power.”
According to the researchers, the stability of an electrolyte determines how many charging and discharging cycles a battery can withstand before losing power. Because of this, scientists are in a race to find the most stable electrolyte materials.
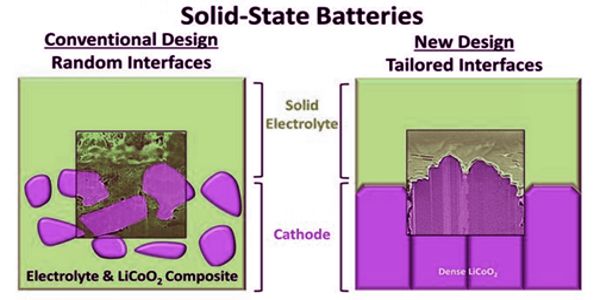
“In the rush to find stable solid electrolyte materials, developers have lost sight of the importance of what is happening at that very thin interface between electrolyte and electrode,” Zahiri said. “However, the stability of the electrolyte is meaningless if the connection between it and the electrodes cannot be evaluated efficiently.”
They discovered a linear relationship between capacity fade and interfacial resistance in dense cathodes, revealing a direct impact of interface crystallography and morphology on solid-state battery performance. Conventional composite cathodes with random crystallographic orientation and a larger interfacial area, on the other hand, exhibited unpredictable behavior.
In the lab, the researchers created electrodes with specific atomic arrangements of sodium and lithium ions. In both lithium and sodium-based solid-state batteries, they discovered correlations between battery performance and interface atomic arrangement. They also discovered that controlling the atomic alignment of the electrodes and minimizing the interface surface area are critical to understanding the nature of interface instabilities and improving cell performance.
“This is a new paradigm for evaluating all of the important solid electrolytes available today,” Cook explained. “Previously, we were largely speculating on which electrode-solid electrolyte interface structures provided the best performance, but now we can test this and determine the best combination of materials and atomic orientations.”
Having this level of control, as demonstrated by co-author mechanical science and engineering professor Elif Ertekin and her group, provided the researchers with the information needed to run atomic simulations, which they hypothesize will lead to even better electrolyte materials in the future, the researchers said.
“We believe this will teach us a lot about investigating emerging solid electronics,” Braun said. “We are not attempting to develop new solid electrolytes; the materials world is already doing an excellent job in this area. Our methodology will enable others to precisely measure the interfacial properties of their new materials, which were previously difficult to determine.”