Electrocatalysis is a process in which an electrode material is used to catalyze an electrochemical reaction. The use of atomic force microscopy (AFM) in electrocatalysis research allows for the direct observation of the electrode surface at the nanoscale, which can provide insight into the fundamental mechanisms of the catalytic process.
A further advancement in atomic force microscopy now allows for the simultaneous imaging of the height profile of nanometre-fine structures, as well as the electric current and frictional force at solid-liquid interfaces. A team from the Helmholtz-Zentrum Berlin (HZB) and the Max Planck Society’s Fritz Haber Institute (FHI) has succeeded in analyzing electrocatalytically active materials and gaining insights that will aid in the optimization of catalysts. The method could also be used to investigate processes on battery electrodes, photocatalysis, or active biomaterials.
It will also be critical to develop cheap and efficient materials that can be used to split water or CO2 via electrocatalysis in order to manage the energy transition. Part of the electrical energy is stored in the chemical reaction products during this process. The nature of the electrode-electrolyte interfaces, i.e. the interfaces between the solid electrodes and the typically aqueous electrolyte, has a large impact on the efficiency of such electrocatalysts. However, spatially resolved physical studies of such solid-liquid interfaces remain uncommon.
We were able to clearly identify islands of copper oxide with higher electrical resistance, as well as grain boundaries and low-conductivity regions in the hydration layer where the catalyst surface comes into contact with the aqueous electrolyte.
Dr Martin Munz
More insights with AFM
Dr. Christopher S. Kley and his colleagues have created a novel approach to correlative atomic force microscopy (AFM). The surface is scanned with an extremely sharp tip, and its height profile is recorded. The force interactions between the tip and the sample surface, including frictional forces, can be measured with high sensitivity by attaching the tip to the end of a miniaturised cantilever. Furthermore, if a voltage is applied, the electrical current flowing through the mechanical contact can be measured.
“This allowed us to determine the electrical conductivity, mechanical-chemical friction, and morphological properties in situ (that is, under the relevant liquid-phase conditions rather than in vacuum or air),” Kley emphasizes.
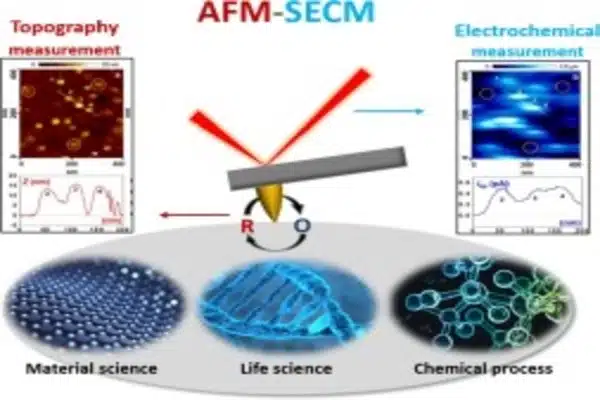
Copper-gold electrocatalyst
Using this method, the scientists investigated a nanostructured and bimetallic copper-gold electrocatalyst in collaboration with Prof. Beatriz Roldán Cuenya of the Fritz-Haber-Institute (FHI). These materials, among others, are used in the electrocatalytic conversion of CO2 into energy carriers. “We were able to clearly identify islands of copper oxide with higher electrical resistance, as well as grain boundaries and low-conductivity regions in the hydration layer where the catalyst surface comes into contact with the aqueous electrolyte,” says Dr Martin Munz, the study’s first author.
Such findings on catalyst-electrolyte interfaces aid in targeted optimization. “We can now see how local electrochemical environments influence charge transfer at the interface,” says Kley.
Focus on solid-liquid interfaces
“However, our findings are of general interest to energy research, particularly in the study of electrochemical conversion processes, which also play a role in battery systems.” Insights into solid-liquid interfaces can also be useful in completely different areas of research, such as understanding corrosion processes, nanosensor systems, and possibly addressing scientific queries in fluidics and environmental sciences, such as dissolution or deposition processes on metal surfaces exposed to water.
This work was completed as part of the CatLab project, in which researchers from the HZB and the FHI of the MPG collaborate to develop thin-film catalysts for the energy transition.