Gravitationally speaking, the universe is a chaotic place. Gravitational waves from unknown sources travel unexpectedly through space, presumably from the early universe.
Scientists have been searching for evidence of these early cosmic gravitational waves, and a team of physicists has recently demonstrated that such waves should have a different signature due to the behavior of quarks and gluons as the cosmos cools. Such a discovery would have a significant impact on which models best represent the universe shortly after the Big Bang. The study was published in the journal Physical Review Letters.
Scientists discovered direct evidence for gravitational waves in 2015 at the LIGO gravitational wave interferometers in the United States. These are distinct (albeit with tiny amplitude) waves from a specific source, such as the merger of two black holes, that wash past Earth. Such waves cause the 4 km perpendicular arms of the interferometers to change length by minute (but distinct) amounts, which is detected by changes in the ensuing interference pattern when laser beams pass back and forth in the detector’s arms.
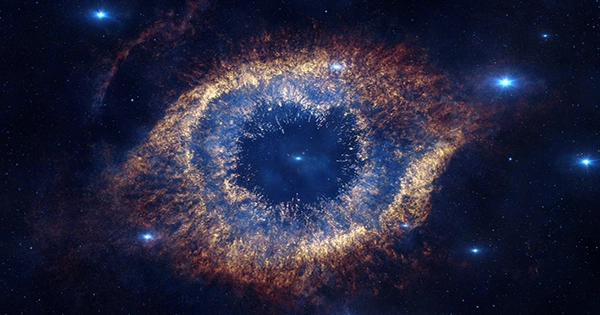
However, there are also smaller gravitational waves, which appear to be noise. Scientists have been assiduously searching for the stochastic gravitational wave background (stochastic meaning randomly determined, i.e. unpredictable). However, because these smaller gravitational waves are more difficult to detect, scientists have turned to millisecond pulsar arrays, in which the distance between Earth and a faraway pulsar serves as the effective interferometer arm length.
Pulsars, which are rotating neutron stars, emit radiation beams, some of which sweep over Earth, much like a lighthouse beam. Pulsars have a remarkably constant period of revolution, and any measurement of their clock timing would be subtly affected by the passing of numerous smaller gravitational waves with light-year wavelengths.
Last year, the NANOgrav collaboration and other researchers revealed evidence that low-frequency, stochastic gravitational waves occur in the spacetime background. But what is their source? Is the backdrop caused by astronomical phenomena such as hundreds of thousands of merging supermassive black holes, supernovae, and so on?
Perhaps the background started in the early cosmos and has been propagating ever since, similar to the cosmic microwave background that fills all of space as a result of photons decoupling from electrons 380,000 years after the Big Bang. Or something else?
Distinguishing the circumstances presents difficulties. Our current understanding of the mechanics of supermassive black holes is insufficient to draw definite conclusions. Furthermore, the continuous spectrum of background gravitational waves is dependent on the microscopic specifics of their source, necessitating thorough numerical calculations.
This new work demonstrates how to distinguish early universe waves from those from other sources. Standard model physics—the successful theories of strong, weak, and electromagnetic interactions—should leave a distinct stamp on the measured background that is unaffected by the specific early universe model used.
As the cosmos cooled after the Big Bang, it passed through several phases. One example is photon decoupling after 380,000 years, when the universe cooled enough for electrons to link with protons and create hydrogen atoms, leaving photons adrift.
However, there was an earlier change, or crossover, when unbound quarks and gluons that had created a quark-gluon plasma condensed into individual particles of two or more quarks stuck together as a result of the strong attraction, with gluons trapped beside them.
This “quantum chromodynamics (QCD) crossover” is projected to occur when the cosmos reaches a temperature of roughly one trillion Kelvin, approximately 10-5 seconds after the Big Bang. This translates to an energy of around 100 MeV. (QCD is the theory of strong forces.)
The nanohertz frequencies explored by pulsar timing arrays are found to be of the same order as the detectable low-frequency stochastic gravitational waves in the background. The crossover does not cause the waves, but the sudden decrease in free particle number alters the equation that regulates the state of the cosmos. Before the QCD crossover, gravitation wave sources create a low-frequency signal that is impacted by the change in the equation of state. Researchers claim that signal can now be seen in pulsar timing array data.
“We think that an accurate characterization of the gravitational wave background for different origins is a crucial step to move forward in this exploration,” said Davide Racco, a co-author on the paper from Stanford University’s Institute for Theoretical Physics.
“We highlight a generic and unavoidable feature for a wide range of primordial phenomena that we prove to be a useful ingredient to discriminate between different sources of the background.”
Such a result would have a surprising influence on the cosmos we observe today, demonstrating how particle physics and cosmology intersect.