Light is important not only as an information carrier for optical computer chips but also for the next generation of quantum computers. The precise control of its interaction with other light and its lossless guidance around sharp corners on tiny chips are the focus of research around the world. In a ‘topological photonic crystal,’ scientists have now demonstrated the spatial confinement of a light wave to a point smaller than the wavelength.
These are man-made electromagnetic materials that allow for the robust manipulation of light. Special properties protect the state, which is important for use in quantum chips, for example. The findings have been published in the prestigious journal “Science Advances.”
Topological crystals work on the basis of specific structures whose properties are largely unaffected by disturbances and deviations. While the effects required for light manipulation are fragile in normal photonic crystals and can be affected by defects in the material structure, topological photonic crystals are protected from this. Topological structures enable properties such as unidirectional light propagation and increased robustness for guiding photons, which are small particles of light – features critical for future light-based technologies.
Photonic crystals influence the propagation of electromagnetic waves by using an optical bandgap for photons, which prevents light from moving in certain directions. With the help of topological photonic crystals, we can create novel unidirectional waveguides that transmit light without back reflection even in the presence of arbitrarily large disorders.
Professor Thomas Zentgraf
Photonic crystals influence the propagation of electromagnetic waves by using an optical bandgap for photons, which prevents light from moving in certain directions. Scattering is common, with some photons reflected back and others reflected away. You can avoid this by using topological light states that span a wide range of photonic crystals. The back reflection is a major issue in standard optical waveguides and fibers because it causes unwanted feedback. Large-scale integration in optical chips, where photons transmit information, is hampered by loss during propagation.
“With the help of topological photonic crystals, we can create novel unidirectional waveguides that transmit light without back reflection even in the presence of arbitrarily large disorder,” explains Professor Thomas Zentgraf, head of Paderborn University’s Ultrafast Nanophotonics research group. The concept, which has its roots in solid-state physics, has already resulted in a plethora of applications, including robust light transmission, topological delay lines, topological lasers, and quantum interference.
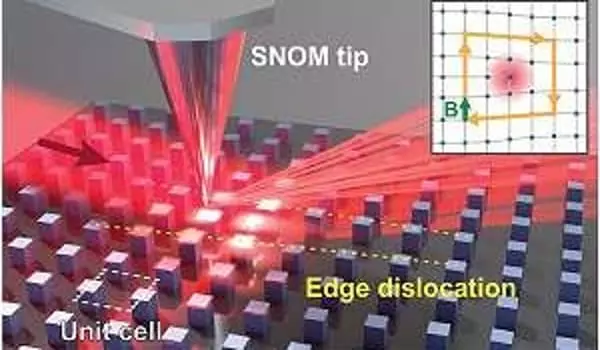
“It was recently demonstrated that topological photonic crystals based on a weak topology with a crystal dislocation in the periodic structure exhibit these special properties as well as topologically-protected strongly spatially localized light states. When something is topologically protected, changes in parameters have no effect on the protected properties. Localized light states are extremely useful for nonlinear amplification, miniaturization of photonic components, and photonic quantum chip integration “Zentgraf adds. In this context, weak topological states are special states of light that result not only from the topological band structure but also from crystal structure formation.
Researchers from Paderborn University and RWTH Aachen University collaborated to demonstrate the existence of such strongly localized light states in topological structures using a special near-field optical microscope. “We demonstrated that the versatility of weak topology can produce a strongly spatially localized optical field in an intentionally induced structural dislocation,” explains Jinlong Lu, the paper’s lead author and a Ph.D. student in Zentgraf’s group. “Our research demonstrates a feasible strategy for achieving a topologically protected, localized zero-dimensional state for light,” Zentgraf adds. The researchers’ findings show that near-field microscopy can be used to characterize topological structures with nanoscale resolution at optical frequencies.
The findings lay the groundwork for the application of strongly localized optical light states based on weak topology. As a result, phase-change materials with tunable refractive indexes could be used for the nanostructures used in the experiment to produce robust and active topological photonic elements. “We’re now working on concepts to equip the dislocation centers in the crystal structure with special quantum emitters for single-photon generation,” Zentgraf says, adding that “these could then be used in future optical quantum computers, where single-photon generation is important.”