In an experiment similar to stop-motion photography, scientists isolated an electron’s intense movement while “freezing” the motion of the much bigger atom it orbits in a sample of liquid water.
The discoveries, published in the journal Science, provide a new window into the electrical structure of liquid-phase molecules on a timescale that X-rays could not reach before. The new technology displays the immediate electronic response when an X-ray hits a subject, which is a crucial step toward understanding how radiation affects objects and humans.
“The chemical reactions induced by radiation that we want to study are the result of the target’s electronic response that happens on the attosecond timescale,” said Linda Young, the study’s senior author and Distinguished Fellow at Argonne National Laboratory.
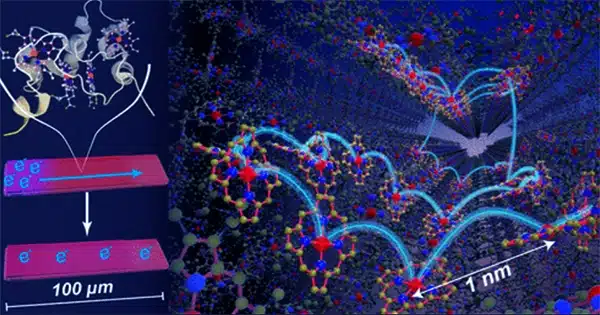
“Until now, radiation chemists could only resolve events on the picosecond timeframe, which is a million times slower than the attosecond. It’s similar to stating, ‘I was born and then died.’ You want to know what happens in between. That’s what we can currently do.
A multi-institutional team of scientists from various Department of Energy national laboratories and universities in the United States and Germany combined experiments and theory to disclose in real time the effects of ionizing radiation from an X-ray source on matter.
Working on the time scales at which the activity occurs will help the study team gain a better understanding of complex radiation-induced chemistry. Indeed, these experts initially collaborated to create the instruments required to evaluate the effects of long-term ionizing radiation exposure on the compounds contained in nuclear waste.
“Members of our early-career network participated in the experiment, and then joined our full experimental and theoretical teams to analyze and understand the data,” said Carolyn Pearce, director of IDREAM EFRC and PNNL chemist. “We couldn’t have done this without the IDREAM partnerships.”
From the Nobel Prize to the field
Subatomic particles move so quickly that catching their motions necessitates a probe capable of measuring time in attoseconds, a time frame so minuscule that there are more attoseconds in one second than there have been seconds in the history of the universe.
The current inquiry draws on the emerging science of attosecond physics, which has been awarded the 2023 Nobel Prize in Physics. Attosecond X-ray pulses are only available in a small number of specialized facilities globally. This research team carried out their experiments at the Linac Coherent Light Source (LCLS), which is part of the SLAC National Accelerator Laboratory in Menlo Park, California. The local team pioneered the construction of attosecond X-ray free electron lasers.
“Attosecond time-resolved experiments are one of the flagship R&D developments at the Linac Coherent Light Source,” said Ago Marinelli of the SLAC National Accelerator Laboratory, who, along with James Cryan, led the development of the synchronized pair of X-ray attosecond pump/probe pulses used in this experiment. “It’s exciting to see these developments being applied to new kinds of experiments and taking attosecond science into new directions.”
The approach created in this work, termed X-ray attosecond transient absorption spectroscopy in liquids, enabled scientists to “watch” electrons powered by X-rays as they moved into an excited state, all before the bulkier atomic nucleus had a chance to shift. They chose liquid water as the test case for their experiment.
“We now have a tool where, in principle, you can follow the movement of electrons and see newly ionized molecules as they’re formed in real-time,” said Young, a professor in the Department of Physics and James Franck Institute at the University of Chicago.
These recently revealed findings settle a long-standing scientific disagreement over whether X-ray signals observed in prior tests are the result of various structural forms, or “motifs,” of water or hydrogen atom movements. These investigations firmly show that the signals do not correspond to two structural motifs in ambient liquid water.
“What people were seeing in previous experiments was the blur caused by moving hydrogen atoms,” he explained. “We were able to eliminate that movement by doing all of our recordings before the atoms had time to move.”