Researchers have created a superconducting nanowire that would allow reliable, easy-to-use electronics. The progress could improve quantum computing, as well as magnetic sensors for brain imaging and telescope applications. Superconductors – materials that conduct electricity without resistance – are exceptional. They offer a macroscopic insight at quantum phenomena, which are typically only detectable at the atomic level. Beyond their physical peculiarity, superconductors are useful as well. They are used in medical imaging, quantum computers, and telescope cameras.
New technology could boost superconducting electronics. The advance could boost quantum computing, as well as magnetic sensors for applications in brain imaging and telescopes.
But the superconducting systems can be finicky. They are also costly to produce and susceptible to err from ambient noise. This may improve, thanks to studies by Karl Berggren’s group in the Department of Electrical Engineering and Computer Science. Researchers are designing a superconducting nanowire that would make more powerful superconducting electronics. The future advantages of nanowires are extracted from their simplicity, Berggren says. “At the end of the day, it’s just a wire.”
Berggren will present a summary of the research at this month’s IEEE Solid-state Circuits Conference.
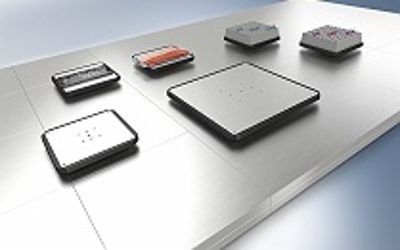
Resistance is futile
Many metals lose resistance and become superconductive at very low temperatures, typically only a few degrees above absolute zero. They are used to detect magnetic fields, particularly in highly sensitive contexts such as brain activity monitoring. They still have uses for both quantum and classical computation.
Many of these superconductors are based on a system developed in the 1960s called the Josephson junction-essentially two superconductors separated by a thin insulator. “That’s what led to conventional superconducting electronics, and then ultimately to the superconducting quantum computer,” Berggren says.
However, the junction of Josephson “is fundamentally quite a delicate object,” Berggren continues. This translates directly into the expense and sophistication of the manufacturing process, particularly for thin insulation later on. Josephson’s junction-based superconductors can also not play well with others: “If you try to interface it with conventional electronics, like the kinds in our phones or computers, the noise from those just swamps the Josephson junction. So, this lack of ability to control larger-scale objects is a real disadvantage when you’re trying to interact with the outside world.”
To overcome these disadvantages, Berggren is developing a new technology – the superconducting nanowire – with roots older than the Josephson junction itself.
Cryotron reboot
In 1956, MIT electrical engineer Dudley Buck published a description of a superconductive device switch called the cryotron. The system was nothing more than two superconducting wires: one was parallel, and the other was coiled around. The cryotron functions as a lever, and when the current flows through the coiled wire, the magnetic field decreases the current flowing through the straight wire.
At the time, the cryotron was significantly simpler than other types of electronic switches, such as vacuum tubes or transistors, and Buck felt that the cryotron could become the building block of computers. But in 1959, Buck died unexpectedly at the age of 32, delaying the production of the cryotron. (Since then, transistors have been scaled to microscopic sizes and are now the central logic elements of computers.)
Now, Berggren is reawakening Buck’s theories about superconducting device switches. “The devices we’re making are very much like cryotrons in that they don’t require Josephson junctions,” he says. In tribute to Buck, he named his superconducting nanowire technology a nano-cryotron-though it functions a little differently from the original cryotron.
The nano-cryotron uses heat to activate a transition instead of a magnetic field. In Berggren’s unit, the current passes through a superconducting, supercooled wire called the “channel.” The channel is intersected by an even smaller wire called the “choke”—like a multi-lane highway intersected by a side path. When the current is sent through the shock, the superconductivity breaks down and heats up. Once the heat travels from the choke to the main channel, it also allows the main channel to lose its superconducting state.
Berggren’s group has already shown proof of concept for the use of nano-cryotrons as an electronic component. Adam McCaughan, a former Berggren student, has created a system that uses nano-cryotrons to add binary digits. And Berggren has successfully used nano-cryotrons as an interface between superconducting instruments and traditional, transistor-based electronics.
Berggren says that his group’s superconducting nanowire could one day supplement or compete with Josephson’s junction-based superconducting systems. “Wires are relatively easy to make, so it may have some advantages in terms of manufacturability,” he says. He thinks that the nano-cryotron will one day find a home in superconducting quantum computers and supercooled telescope electronics. Wires have low power dissipation, but they can also be handy for energy-hungry applications, he notes. “It’s probably not going to replace the transistors in your phone, but if it could replace the transistor in a server farm or data center? That would be a huge impact.”
Beyond special uses, Berggren takes a general view of his work on superconducting nanowires. “We’re doing fundamental research, here. While we’re interested in applications, we’re just also interested in: What are some different kinds of ways to do computing? As a society, we’ve really focused on semiconductors and transistors. But we want to know what else might be out there.”