On a quantum computer, quantum physicists successfully replicated superdiffusion in a system of interacting quantum particles. This is the first step toward doing extremely difficult quantum transport computations on quantum hardware, and such work promises to provide new light on condensed matter physics and materials research as the technology improves over time.
Trinity’s quantum physicists, in partnership with IBM Dublin, have successfully simulated super diffusion on a quantum computer in a system of interacting quantum particles. This is the first step toward doing extremely difficult quantum transport computations on quantum hardware, and such work promises to provide new light on condensed matter physics and materials research as the technology improves over time.
The work is one of the first outputs of the TCD-IBM predoctoral scholarship programme which was recently established where IBM hires PhD students as employees while being co-supervised at Trinity. The paper was published recently in the leading Nature journal NPJ Quantum Information.
In general, conventional computers face a formidable challenge in simulating the dynamics of a complex quantum system with many interacting constituents. Consider the 27 qubits available on this device. In quantum physics, the state of such a system is theoretically defined by an object known as a wave function.
Professor John Goold
In the promising subject of quantum computation, IBM is a global leader. The early-stage quantum computer employed in this work is made up of 27 superconducting qubits (qubits are the basic building blocks of quantum logic) and is physically located in IBM’s lab in Yorktown Heights, New York, but is programmed remotely from Dublin.
Quantum computing is now one of the most interesting technologies, with commercial applications likely within the next decade. Aside from commercial uses, quantum computers can help with some fascinating basic concerns. One such question involving quantum simulation was addressed by a team from Trinity and IBM Dublin.
Explaining the significance of the work and the idea of quantum simulation in general, Trinity’s Professor John Goold, Director of the newly established Trinity Quantum Alliance, who led the research, explains:
“In general, conventional computers face a formidable challenge in simulating the dynamics of a complex quantum system with many interacting constituents. Consider the 27 qubits available on this device. In quantum physics, the state of such a system is theoretically defined by an object known as a wave function. To depict this object using a conventional computer, a massive number of coefficients must be maintained in memory, and the demands rise exponentially with the number of qubits; around 134 million coefficients in the case of this simulation.
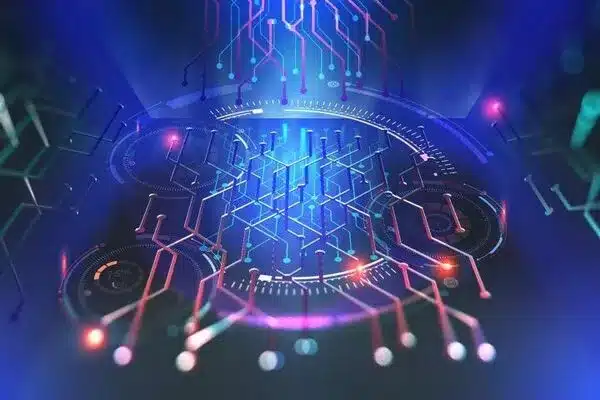
“To describe a system with 300 qubits, you would need more coefficients than there are atoms in the observable universe, and no classical computer will be able to precisely capture the system’s state. In other words, while modelling quantum systems, we strike a brick wall. The concept of employing quantum systems to simulate quantum dynamics dates back to the Nobel Prize-winning American physicist Richard Feynman, who claimed that quantum systems can be best simulated using quantum systems. The explanation is simple: you naturally take advantage of the fact that the quantum computer is described by a wave function, hence avoiding the requirement for exponential classical resources for state storage.”
So what exactly did the team simulate. Prof. Goold continues:
“Some of the simplest non-trivial quantum systems are spin chains. These are systems of little connected magnets called spins, which mimic more complex materials and are used to understand magnetism. We were interested in a model called the Heisenberg chain and we were particularly interested in the long-time behaviour of how spin excitations are transported across the system. In this long-time limit, quantum many-body systems enter a hydrodynamic regime and transport is described by equations that describe classical fluids.
“We were interested in a specific regime where something called super-diffusion occurs because the underlying physics is governed by the Kardar-Parisi-Zhang equation.” This is an equation that often represents the stochastic growth of a surface or interface, such as how snow accumulates during a snowfall, how a coffee cup stain on cloth accumulates over time, or how a fluff fire accumulates. It is known that the propagation results in superdiffusive transport. This is transportation that becomes faster as the system size increases. It’s incredible that the identical equations that govern these occurrences appear in quantum dynamics, and we were able to validate them using the quantum computer. This was the work’s main accomplishment.”
Nathan Keenan, an IBM-Trinity predoctoral fellow who programmed the device as part of the study, discusses some of the complexities of programming quantum computers.
“Performance of useful calculations in the presence of noise is the biggest problem with programming quantum computers,” he stated. “The chip-level operations are imperfect, and the computer is extremely sensitive to disturbances from its laboratory environment.” As a result, you want to keep the runtime of a useful software as short as possible, as this reduces the time that these mistakes and disturbances can occur and damage your outcome.”