According to a new study from Weill Cornell Medicine experts, a protein that controls how DNA is wrapped within chromosomes plays an important role in the optimal functioning of blood stem cells, which produce all blood cells in the body.
In plants, mammals, and most other species, the protein known as histone H3.3 organizes the spool-like structures around which DNA is coiled. Histones allow DNA to be firmly packed and act as platforms for minor chemical modifications known as epigenetic modifications, which can loosen or tighten the wrapped DNA to influence local gene activity.
The study, published in Nature Cell Biology, looked at the role of H3.3 in blood stem cells, also known as hematopoietic stem cells (HSCs), which are a significant focus of attempts to produce stem-cell-based therapeutics. Most HSCs normally remain in a stem-like, uncommitted form where they can live long-term, slowly self-renewing, however some HSCs develop or “differentiate” to create all of the different lineage-specific blood cell types. The study discovered that H3.3 is essential for both processes; removing the protein from HSCs resulted in decreased HSC survival, an imbalance in the types of blood cells produced by the HSCs, and other problems.
Most importantly, we discovered evidence that H3.3 affects HSCs in part by anchoring several key epigenetic marks at developmental genes and endogenous retroviruses (ERVs), which are remnants of viruses that once inscribed themselves into the DNA of our distant evolutionary ancestors.
Dr. Shahin Rafii
“How hematopoietic stem cells coordinate their self-renewal and differentiation into various blood cell types in a balanced way has been a mystery to a great extent, but this study helps us understand those processes much better at the molecular level and gives us many new clues to pursue in further investigations,” said study co-senior author Dr. Shahin Rafii, director of the Ansary Stem Cell Institute, chief of the Division of Regenerative Medicine and the Arthur B. Belfer Professor in Genetic Medicine at Weill Cornell Medicine.
The study was a collaboration that also included co-first and co-senior authors Dr. Ying Liu and Dr. Peipei Guo, who are senior instructors in the Rafii Laboratory; co-senior author Dr. Duancheng Wen, assistant professor of reproductive medicine research in obstetrics and gynecology; and co-author Dr. Steven Josefowicz, assistant professor of pathology and laboratory medicine and a member of the Sandra and Edward Meyer Cancer Center, all of Weill Cornell Medicine.
Because of their relevance in health and disease, as well as their promise in regenerative therapy, HSCs are among the most studied stem cells. A single HSC can give rise to all types of blood cells, including red blood cells and platelets, as well as T cells, B cells, and pathogen-engulfing macrophages. A deeper understanding of how HSCs function could lead to a variety of applications, such as lab-grown blood for transfusions and improved HSC transplants for cancer patients. Furthermore, understanding how HSCs, when mutated, give rise to leukemias could lead to the development of new therapeutics for these often-refractory malignant diseases.
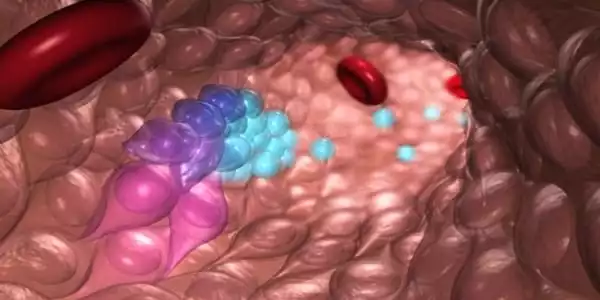
H3.3 has also become a key subject of study for biologists in recent years, as proof of its involvement in HSCs and other stem cells – as well as its function in many malignancies when mutated – has accumulated. However, it is unclear exactly what histone H3.3 performs in HSCs and other cell types where it is found.
“The fact that two distinct genes (H3.3A and H3.3B) code for the identical H3.3 protein adds to the project’s complication. As a result, we had to methodically remove both genes in mice by genetic engineering, a tremendous task that necessitated extensive genetic manipulation of stem cells” According to Dr. Wen.
“Our strong mouse model enables inducible and full deletion of the H3.3 protein in all organs, or certain types of organs, at various embryonic stages of a mouse,” said Dr. Liu, who is also a research associate in Dr. Rafii’s lab. “We demonstrated that the absence of H3.3 in adulthood predominantly causes a loss of the long-term, self-renewing HSCs on which future blood-cell generation is dependent.” Simultaneously, afflicted HSCs developed into mature blood cell types with an aberrant skew or bias toward specific types of white blood cells, such as granulocytes and macrophages.
“Most importantly, we discovered evidence that H3.3 affects HSCs in part by anchoring several key epigenetic marks at developmental genes and endogenous retroviruses (ERVs),” she continued, “which are remnants of viruses that once inscribed themselves into the DNA of our distant evolutionary ancestors.”
“One intriguing observation was that H3.3’s deletion caused the loss of epigenetic marks that normally suppress ERVs, which in turn activated an inflammatory response in affected cells, and then drove the cells’ skewed production of blood cell types — a skew similar to what is seen in some leukemias,” said Dr. Guo, who is also a research associate in Dr. Rafii’s laboratory.
“H3.3 appears to be working as a master regulator of self-renewal and differentiation in HSCs — which is wild, and suggests at a very broad potential as a therapeutic target eventually,” said co-author Andrew Daman, a doctoral candidate in the Josefowicz group at Weill Cornell Medical College.
“Our take-home message is that normal blood cell formation necessitates adequate epigenetic regulation, which H3.3 provides,” Dr. Liu added.
The researchers are now planning additional investigations in HSCs and other cell types to learn more about how H3.3 works and what happens when it is missing. More importantly, finding methods to monitor the H3.3 command of the epigenetic landscape may allow them to promote blood production more effectively. “Finally, our team is looking at how H3.3 regulates the function of nurturing niche cells, such as blood vessels, that orchestrate stem cell self-renewal and may prevent the emergence of malignancies like leukemias,” said Dr. Rafii, who is also a member of the Meyer Cancer Center.