A quantum simulator is providing physicists with a clear picture of spin-charge separation, a strange phenomenon in which two parts of indivisible particles known as electrons travel at different speeds in extremely cold 1D wires. The findings have implications for quantum computing and atom-scale wire electronics.
Rice University’s quantum simulator is providing physicists with a clear view of spin-charge separation, the quantum world’s version of the magician’s illusion of sawing a person in half. Electrons are subatomic particles that cannot be separated. Regardless, quantum mechanics dictates that two of their properties, spin and charge, travel at different speeds in one-dimensional wires.
Rice physicists Randy Hulet, Ruwan Senaratne and Danyel Cavazos built an ultracold venue where they could repeatedly view and photograph a pristine version of this quantum spectacle, and they collaborated with theorists from Rice, China, Australia and Italy on the published results.
Quantum simulators use the quantum properties of real-world objects such as atoms, ions, or molecules to solve problems that conventional computers find difficult or impossible to solve. Rice’s spin-charge simulator substitutes lithium atoms for electrons and a light channel for a 1D electronic wire.
The universe is awash in heat, which obscures atoms’ quantum behavior. Hulet’s team used laser cooling to make its atoms 1 million times colder than the coldest natural object in the universe in order to detect quantum effects in lithium. Additional lasers were used to create the 1D light channel, also known as an optical waveguide.
People have observed spin-charge separation in solid-state materials, but not in a very clean or quantitative way. Our experiment is the first to provide quantifiable measurements that can be compared to a nearly exact theory.
Randy Hulet
Ideal made real
Electrons are antisocial quantum particles that refuse to coexist in the same space. In 1D, spin-charge separation is a manifestation of that mutual hatred. It was theoretically developed around 60 years ago by physicists Shinichiro Tomonaga and Joaquin Luttinger. However, measuring it in electronic materials has proven extremely difficult.
Hulet, Rice’s Fayez Sarofim Professor of Physics and a member of the Rice Quantum Initiative, believes the simulator will allow researchers to investigate the physics of spin-charge separation in ways that were previously impossible.
“People have observed spin-charge separation in solid-state materials, but not in a very clean or quantitative way,” Hulet explained. “Our experiment is the first to provide quantifiable measurements that can be compared to a nearly exact theory.”
Real materials have flaws, but Tomonaga and Luttinger’s theory describes electron behavior in a flawless 1D wire. The new simulation reveals the behavior of real quantum particles in a pristine environment similar to the theoretical ideal. “Cold atoms allow us to tune the strength of the interaction between particles, allowing for an almost textbook comparison with Tomonaga-Luttinger Liquid theory,” Hulet said.
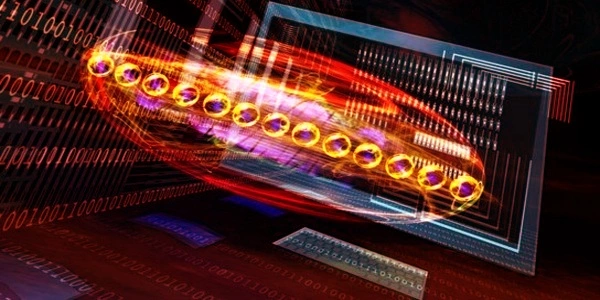
Fewer dimensions, different physics
When one electron strikes another, it imparts energy that can excite the struck electron to a higher energy state. In a 3D material, the excited electron zings away, collides with something, loses a bit of energy, caroms off in a new direction to collide with something else and so on. But that cannot happen in 1D.
“In 1D, every excitation is collective,” Hulet said. “If you push an electron in a 1D wire, it pushes on the one next to it, and it pushes on the one next to it, and so on.”
Senaratne, a researcher in Hulet’s lab, stated, “They are unable to move around one another. They’re stuck in a line. If you move one of them, you must move all of them. As a result, electron excitations in a 1D wire must be collective.”
Excitations ripple down the wire in waves when electrons collide in 1D. Tomonaga and Luttinger realized that spin excitation waves would move more slowly than charge waves. However, Hulet claims that imagining this separation as the splitting of an electron or, in the case of the simulator, the splitting of a lithium atom is incorrect.
“It is not intuitive,” he said. “You have to envision matter existing as waves.”
Comparing speeds
Hulet’s group created a 1D simulator in 2018 that could excite the equivalent of charge waves, and his team measured how fast the waves moved. They needed to compare the speed of those charge waves with the speed of spin waves moving down the line to test the Tomonaga-Luttinger Liquid model.
“At the time, we couldn’t excite spin waves, but Ruwan and Danyel put together a system that could,” Hulet explained. “We had to overcome a technical stumbling block related to a process known as spontaneous emission.”
Cavazos stated: “The effect we’re looking for is a little subtle. So if you disturb it too much, it will simply wash away. An analogy would be if we were attempting to photograph something but the flash was causing damage to the subject. In this analogy, we had to change the color of the flash to make it gentler. We also tweaked the system slightly to make it less fragile than before. That combination enabled us to see the subtle effect.”
The experimental data closely matched predictions from a state-of-the-art theoretical calculation made by the research groups of study co-author Xi-Wen Guan at both the Chinese Academy of Sciences and Australian National University and of co-author Han Pu at Rice.
1D matters
“As integrated circuits become smaller, chipmakers have to start worrying about dimensionality,” Hulet said. “Their circuits eventually become a one-dimensional system that has to conduct and transport electrons in the same way as the one-dimensional wires we’ve been talking about.”
The research could also help with the development of technology for topological quantum computers, which would encode information in qubits without the decoherence that plagues today’s quantum computers. Microsoft and others hope to create topological qubits using Majorana fermions, which may exist in some 1D or 2D superconductors. Hulet’s long-term goal is to simulate a type of 1D superconductor capable of hosting Majorana fermions, and this week’s report represents a significant step toward that goal, according to Hulet.
“We’re learning about these systems as we go,” he explained. “It is critical for someone to be doing the fundamentals, learning how to manipulate things experimentally, what the observations mean, and how to understand them. This work is a significant step forward. It demonstrates our ability to conduct experiments on a system that simulates a one-dimensional superconductor.”