Researchers have created a novel superconducting qubit architecture that can perform operations between qubits with far greater precision than scientists have previously achieved. This architecture, which makes use of a relatively new type of superconducting qubit known as fluxonium, is scalable and could be used to build a large-scale quantum computer in the future.
Quantum computers may be able to solve problems that are far too complex for today’s most powerful supercomputers in the future. Quantum versions of error correction codes must be able to account for computational errors faster than they occur in order to fulfill this promise. However, quantum computers are not yet robust enough to perform error correction at commercially relevant scales.
On their way to overcoming this barrier, MIT researchers demonstrated a novel superconducting qubit architecture that can perform operations between qubits – the building blocks of a quantum computer – with far greater accuracy than scientists have previously been able to achieve.
They use fluxonium, a relatively new type of superconducting qubit with a much longer lifespan than more commonly used superconducting qubits. Their architecture includes a special coupling element between two fluxonium qubits that enables them to perform highly accurate logical operations known as gates. It eliminates a type of unwanted background interaction that can cause errors in quantum operations.
These findings are immediately applicable and have the potential to change the state of the entire field. We are confident that this architecture, or something similar using fluxonium qubits, holds great promise for actually building a useful, fault-tolerant quantum computer.
Dr. Kannan
This approach enabled two-qubit gates that exceeded 99.9 percent accuracy and single-qubit gates with 99.99 percent accuracy. In addition, the researchers implemented this architecture on a chip using an extensible fabrication process.
“Building a large-scale quantum computer starts with robust qubits and gates. We showed a highly promising two-qubit system and laid out its many advantages for scaling. Our next step is to increase the number of qubits,” says Leon Ding PhD ’23, who was a physics graduate student in the Engineering Quantum Systems (EQuS) group and is the lead author of a paper on this architecture.
Ding wrote the paper with Max Hays, an EQuS postdoc; Youngkyu Sung Ph.D. ’22; Bharath Kannan Ph.D. ’22, who is now CEO of Atlantic Quantum; Kyle Serniak, a staff scientist and team lead at MIT Lincoln Laboratory; and senior author William D. Oliver, the Henry Ellis Warren professor of electrical engineering and computer science and of physics, director of the Center for Quantum Engineering, leader of EQuS, and associate director of the Research Laboratory of Electronics; as well as others at MIT and MIT Lincoln Laboratory. The research appears today in Physical Review X.
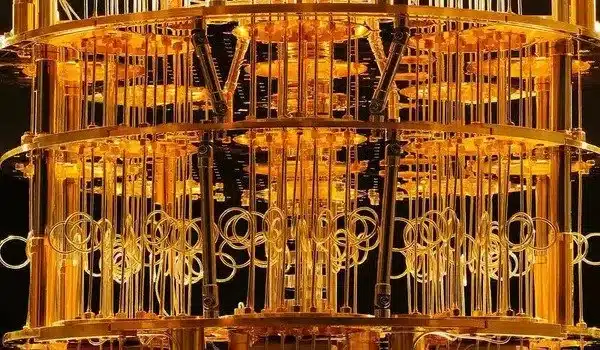
A new take on the fluxonium qubit
Gates in a traditional computer are logical operations performed on bits (a series of 1s and 0s) that allow computation. Gates in quantum computing are conceptually similar: a single qubit gate is a logical operation on one qubit, whereas a two-qubit gate is an operation that depends on the states of two connected qubits.
The accuracy of quantum operations performed on these gates is measured by fidelity. Because quantum errors accumulate exponentially, gates with the highest fidelities are required. With billions of quantum operations taking place in a large-scale system, even minor errors can quickly cause the entire system to fail.
In practice, error correcting codes would be used to achieve such low error rates. However, the operations must meet a “fidelity threshold” in order to implement these codes. Furthermore, exceeding this threshold reduces the overhead required to implement error-correcting codes.
Transmon qubits have been used primarily by researchers in their efforts to build quantum computers for more than a decade. A fluxonium qubit, a more recent type of superconducting qubit, was invented. Fluxonium qubits have longer lifetimes, or coherence times, than transmon qubits. Coherence time is a measure of how long a qubit can perform operations or run algorithms before losing all of its information.
“The longer a qubit lives, the higher fidelity the operations it tends to promote. These two numbers are tied together. But it has been unclear, even when fluxonium qubits themselves perform quite well, if you can perform good gates on them,” Ding says.
For the first time, Ding and his collaborators found a way to use these longer-lived qubits in an architecture that can support extremely robust, high-fidelity gates. In their architecture, the fluxonium qubits were able to achieve coherence times of more than a millisecond, about 10 times longer than traditional transmon qubits.
“Over the last couple of years, there have been several demonstrations of fluxonium outperforming transmons on the single-qubit level,” says Hays. “Our work shows that this performance boost can be extended to interactions between qubits as well.”
The fluxonium qubits were developed in a close collaboration with MIT Lincoln Laboratory, (MIT-LL), which has expertise in the design and fabrication of extensible superconducting qubit technologies.
“This experiment was exemplary of what we call the ‘one-team model’: the close collaboration between the EQuS group and the superconducting qubit team at MIT-LL,” says Serniak. “It’s worth highlighting here specifically the contribution of fabrication team at MIT-LL — they developed the capability to construct dense arrays of more than 100 Josephson junctions specifically for fluxoniums and other new qubit circuits.”
A stronger connection
Their novel architecture involves a circuit that has two fluxonium qubits on either end, with a tunable transmon coupler in the middle to join them together. This fluxonium-transmon-fluxonium (FTF) architecture enables a stronger coupling than methods that directly connect two fluxonium qubits.
FTF also reduces unwanted background interactions that occur during quantum operations. Stronger couplings between qubits, known as static ZZ interactions, can typically result in more of this persistent background noise. However, the FTF architecture addresses this issue.
The researchers were able to demonstrate single-qubit gate fidelity of 99.99 percent and two-qubit gate fidelity of 99.9 percent due to their ability to suppress these unwanted interactions and the longer coherence times of fluxonium qubits. These gate fidelities are significantly higher than the threshold required for certain common error-correcting codes, and they should enable error detection in larger-scale systems.
“Through redundancy, quantum error correction increases system resilience.” We can improve overall system performance by adding more qubits, provided the qubits are individually ‘good enough.’ Consider attempting to complete a task with a classroom full of kindergarteners. “There’s a lot of chaos there, and adding more kindergartners won’t help,” Oliver explains. “However, a group of mature graduate students working together produces results that outperform any one of the individuals — that is the threshold concept.” While there is still much work to be done to create an extensible quantum computer, it begins with high-quality quantum operations that are well above threshold.”
Building off these results, Ding, Sung, Kannan, Oliver, and others recently founded a quantum computing startup, Atlantic Quantum. The company seeks to use fluxonium qubits to build a viable quantum computer for commercial and industrial applications.
“These findings are immediately applicable and have the potential to change the state of the entire field.” This demonstrates to the community that there is another way forward. “We are confident that this architecture, or something similar using fluxonium qubits, holds great promise for actually building a useful, fault-tolerant quantum computer,” Kannan says.
While such a computer is still likely to be 10 years away, he believes this research is an important step in the right direction. The researchers intend to demonstrate the benefits of the FTF architecture in systems with more than two connected qubits in the coming months.