Carbon-based quantum technology is the research and development of quantum technologies based on carbon-based materials such as carbon nanotubes and graphene. Quantum technologies use quantum mechanics concepts to do tasks that are difficult or impossible for classical technology. Carbon-based materials have distinct characteristics that make them intriguing candidates for a wide range of quantum applications.
The characteristics of graphene nanoribbons can be carefully adjusted. Researchers have attached electrodes to individual atomically precise nanoribbons, clearing the path for accurate characterization of the interesting ribbons and their potential use in quantum technology.
Quantum technology is both fascinating and confusing. It is expected to provide us with a variety of technological breakthroughs in the coming decades, including smaller and more precise sensors, highly secure communication networks, and powerful computers capable of developing new drugs and materials, controlling financial markets, and predicting the weather much faster than current computing technology.
This project enables the realization of single nanoribbon devices, not only to study fundamental quantum effects such as how electrons and phonons behave at the nanoscale, but also to exploit such effects for applications in quantum switching, quantum sensing, and quantum energy conversion.
Hatef Sadeghi
To do so, we require quantum materials, which are substances that exhibit strong quantum physical phenomena. Graphene is one such substance. This two-dimensional structural form of carbon possesses remarkable physical features, including extremely high tensile strength, thermal and electrical conductivity, and quantum effects. By further restricting the previously two-dimensional material, for example, by giving it a ribbon-like shape, a variety of controllable quantum effects emerge.
This is precisely what Mickael Perrin’s team leverages in their work: For several years now, scientists in Empa’s Transport at Nanoscale Interfaces laboratory, headed by Michel Calame, have been conducting research on graphene nanoribbons under Perrin’s leadership. “Graphene nanoribbons are even more fascinating than graphene itself,” explains Perrin. “By varying their length and width, as well as the shape of their edges, and by adding other atoms to them, you can give them all kinds of electrical, magnetic, and optical properties.”
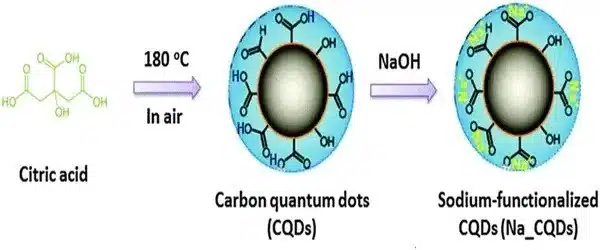
Ultimate precision – down to single atoms
It is difficult to do research on the potential ribbons. The narrower the ribbon, the more evident its quantum features are – but it also gets more difficult to access a single ribbon at a time. This is exactly what needs to be done in order to identify the unique properties and potential applications of this quantum substance from collective effects.
In a recent study published in the journal Nature Electronics, Perrin and Empa researcher Jian Zhang, along with an international team, succeeded for the first time in touching individual long and atomically precise graphene nanoribbons. “A graphene nanoribbon only nine carbon atoms wide measures as little as one nanometer in width,” Zhang explains. To ensure that only a single nanoribbon is contacted, the researchers employed electrodes of a similar size: They used carbon nanotubes that were also only 1 nanometer in diameter.
Precision is key for such a delicate experiment. It begins with the source materials. The researchers obtained the graphene nanoribbons via a strong and long-standing collaboration with Empa’s nanotech surfaces laboratory, headed by Roman Fasel. “Roman Fasel and his team have been working on graphene nanoribbons for a long time and can synthesize many different types with atomic precision from individual precursor molecules,” Perrin explains. The precursor molecules came from the Max Planck Institute for Polymer Research in Mainz.
Interdisciplinarity is essential for advancing the state of the art, and different worldwide research organizations were involved, each bringing their unique specialty to the table: The carbon nanotubes were created by a research group at Peking University, and the Empa researchers teamed with computational scientists from the University of Warwick to analyze the study’s findings. “A project like this would not be possible without collaboration,” says Zhang.
Contacting individual ribbons with nanotubes proved to be a significant problem for the researchers. “The carbon nanotubes and graphene nanoribbons are grown on separate substrates,” explains Zhang. “First, the nanotubes must be moved to the device substrate and made contact with metal electrodes.” Then we separated them into two electrodes using high-resolution electron-beam lithography.” The ribbons are then transferred to the same substrate. Precision is essential: even little rotations of the substrates can drastically limit the likelihood of good contact. “Having access to high-quality infrastructure at the Binnig and Roher Nanotechnology Center at IBM Research in Rüschlikon was essential for testing and implementing this technology,” Perrin explains.
From computers to energy converters
Charge transfer measurements proved the success of the scientists’ experiment. “Because quantum effects are usually more pronounced at low temperatures,” Perrin continues, “we performed the measurements at temperatures close to absolute zero in a high vacuum.” However, he quickly adds another intriguing feature of graphene nanoribbons: “Due to the extremely small size of these nanoribbons, we expect their quantum effects to be so robust that they are observable even at room temperature.” According to the researcher, this could allow us to develop and run devices that actively exploit quantum phenomena without the need for complex cooling infrastructure.
“This project enables the realization of single nanoribbon devices, not only to study fundamental quantum effects such as how electrons and phonons behave at the nanoscale, but also to exploit such effects for applications in quantum switching, quantum sensing, and quantum energy conversion,” adds Hatef Sadeghi, a professor at the University of Warwick who collaborated on the project.