A supernova can happen in one of two ways. Either a massive star will run out of fuel, ceasing to generate fusion energy in its core and collapsing inward under the force of its own gravity to form a neutron star or a black hole, or a white dwarf star will accrete material from a companion star until it reaches a critical mass and explodes.
A supernova remnant (SNR) is the structure left over after a star explodes in a supernova. The supernova remnant is surrounded by an expanding shock wave and is made up of ejected material from the explosion as well as interstellar material that it sweeps up and shocks along the way. A massive star may run out of fuel, ceasing to generate fusion energy in its core and collapsing inward under the force of its own gravity to form a neutron star or a black hole; or a white dwarf star may accrete material from a companion star until it reaches a critical mass and explodes in a thermonuclear explosion.
Shockwaves can propagate through molecular clouds of gas and dust when triggered by an external agent, forming pockets of dense material. That dense gas and dust eventually collapses and begins to form new stars. The interaction was simulated using a high-powered laser and a foam ball. A dense area within a molecular cloud is represented by the foam ball. The high-powered laser generates a blast wave that travels through a gas chamber and into the ball, where the compression was observed using X-ray images.
Molecular clouds are space-based collections of gas and dust. When left alone, the clouds maintain a peaceful equilibrium.
Our primitive molecular cloud, where the sun formed, was most likely triggered by supernova remnants. This experiment paves the way for laboratory astrophysics to understand all of these major points in a new and promising way.
Bruno Albertazzi
Shockwaves can propagate through the gas and dust to create pockets of dense material when triggered by an external agent, such as supernova remnants. That dense gas and dust eventually collapses and begins to form new stars.
Astronomical observations lack the spatial resolution required to observe these processes, and numerical simulations are incapable of handling the complexities of the interaction between clouds and supernova remnants. As a result, the triggering and formation of new stars in this manner remains largely unknown.
In Matter and Radiation at Extremes, by AIP Publishing in partnership with China Academy of Engineering Physics, researchers from the Polytechnic Institute of Paris, the Free University of Berlin, the Joint Institute for High Temperatures of the Russian Academy of Sciences, the Moscow Engineering Physics Institute, the French Alternative Energies and Atomic Energy Commission, the University of Oxford, and Osaka University modeled the interaction between supernova remnants and molecular clouds using a high-power laser and a foam ball.
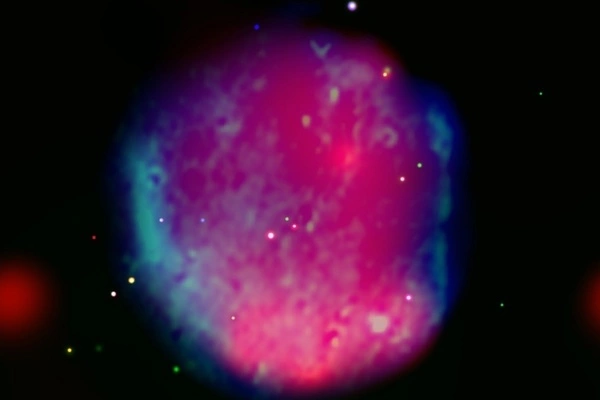
The foam ball represents a dense area within a molecular cloud. The high-power laser creates a blast wave that propagates through a surrounding chamber of gas and into the ball, where the team observed the compression using X-ray images.
“We are really looking at the beginning of the interaction,” said author Bruno Albertazzi. “In this way, you can see if the average density of the foam increases and if you will begin to form stars more easily.”
The mechanisms that cause star formation are fascinating on many levels. They can influence the rate and evolution of a galaxy’s star formation, help explain the formation of the most massive stars, and have consequences in our own solar system.
“Our primitive molecular cloud, where the sun formed, was most likely triggered by supernova remnants,” author Albertazzi explained. “This experiment paves the way for laboratory astrophysics to understand all of these major points in a new and promising way.”
Some of the foam compressed, while some stretched out. Because the stretched mass changed the average density of the material, the authors will need to account for it in the future to truly measure the compressed material and the shockwave’s impact on star formation. They intend to investigate the effects of radiation, magnetic fields, and turbulence.
“The purpose of this first paper was to demonstrate the capabilities of this new platform by introducing a new topic that could be investigated using high-power lasers,” Albertazzi explained.