CRISPR-Cas9 activity visualization was already a well-studied area, with several methods for visualizing the activity of CRISPR genetic scissors. These visualization techniques aimed to shed light on how CRISPR-Cas9 works and how it affects the targeted genes.
Within milliseconds, scientists have developed a new method for measuring the smallest twists and torques of molecules. The method allows researchers to monitor the gene recognition of CRISPR-Cas protein complexes, also known as ‘genetic scissors,’ in real time and at high resolution. The data obtained allows the recognition process to be precisely characterized and modelled in order to improve the precision of the genetic scissors.
When bacteria are attacked by a virus, they can defend themselves with a mechanism that fends off the intruder’s genetic material. The key is CRISPR-Cas protein complexes. Only in the last decade has their role in adaptive immunity in microorganisms been discovered and elucidated. The CRISPR complexes recognise a short sequence in the attacker’s DNA using an embedded RNA.
CRISPR complexes do, on occasion, react to gene segments that differ slightly from the sequence specified by the RNA. This has unfavourable side effects in medical applications. The causes of this are not yet well understood, as the process could not be directly observed until now.
Dominik Kauert
Since then, the mechanism of sequence recognition by RNA has been used to selectively turn off and modify genes in any organism. This discovery revolutionized genetic engineering and was already recognized in 2020 with the Nobel Prize in Chemistry awarded to Emmanuelle Charpentier and Jennifer A. Doudna.
CRISPR complexes do, on occasion, react to gene segments that differ slightly from the sequence specified by the RNA. This has unfavorable side effects in medical applications. “The causes of this are not yet well understood, as the process could not be directly observed until now,” says Dominik Kauert, a Ph.D. student who worked on the project.
One common method of visualization involved the use of fluorescent markers or reporters. Researchers could attach a fluorescent marker to the CRISPR-Cas9 system, allowing them to track its movement and activity in living cells. Scientists could learn a lot about when and where the CRISPR-Cas9 system was active by observing fluorescence patterns.
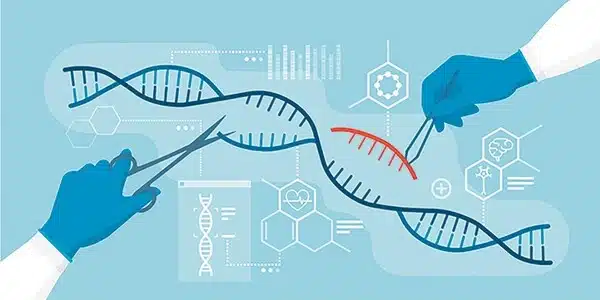
Nanoscale processes tracked in detail
To better understand the recognition process, the team led by Professor Ralf Seidel and Dominik Kauert exploited the fact that the target sequence’s DNA double helix is unwound during recognition to allow base pairing with the RNA. “The central question of the project was therefore whether the unwinding of a piece of DNA that is only 10 nanometres (nm) long could be tracked in real time at all,” says Kauert.
The scientists had to make the unwinding process visible to the microscope in order to observe it in detail. To achieve this goal, the team drew on DNA nanotechnology achievements, which can be used to create any three-dimensional DNA nanostructure. The researchers created a 75 nm long DNA rotor arm with a gold nanoparticle attached to its end using the so-called DNA origami technique. In the experiment, the rotation of the gold nanoparticle along a circle with a diameter of 160 nm was transferred to the unwinding of the 2 nm thin and 10 nm long DNA sequence – this movement can be magnified and tracked using a special microscope setup.
The researchers were able to observe sequence recognition by the CRISPR Cascade complex almost base pair by base pair using this new method. Surprisingly, base pairing with the RNA is not advantageous in terms of energy, implying that the complex is only unstably bound during sequence recognition. Only when the entire sequence is recognized does stable binding occur, after which the DNA is destroyed. If the target sequence is the “wrong” one, the process is aborted.
Findings will help in selecting suitable RNA sequences
The fact that the recognition process sometimes produces incorrect results is due to its stochastic nature, i.e. random molecular movements, as the researchers have now demonstrated. “Thermal fluctuations in base pairing drive sequence recognition,” says Kauert. Using the data collected, a thermodynamic model of sequence recognition that describes the recognition of deviating sequence segments was developed. In the future, this should allow for better selection of RNA sequences that recognize only the desired target sequence, thereby improving the precision of genetic manipulation.
Because the designed nanorotors are universal in their suitability for measuring twists and torques in single molecules, they can also be used for other CRISPR-Cas complexes or biomolecules.