The James Webb Space Telescope (JWST) is expanding scientists’ understanding of planet formation by studying their birthplaces and the circumstellar disks that encircle young stars.
In a paper published in The Astronomical Journal, a team of scientists led by Naman Bajaj of the University of Arizona and including Dr. Uma Gorti from the SETI Institute imaged for the first time winds from an old planet-forming disk (still very young relative to the sun) that is actively dispersing its gas content. The disk has been imaged previously, but winds from older disks have not. Knowing when the gas disperses is crucial because it limits the time available for fledgling planets to consume gas from their surroundings.
The discovery is based on the study of TCha, a young star (relative to the sun) surrounded by an eroding disk known for its large dust gap, which is about 30 astronomical units in radius. Astronomers have photographed the dispersing gas (also known as winds) for the first time using the four noble gas lines neon (Ne) and argon (Ar), one of which is the first observation in a planet-forming disk. The photos of [Ne II] demonstrate that the wind is coming from a large area of the disk.
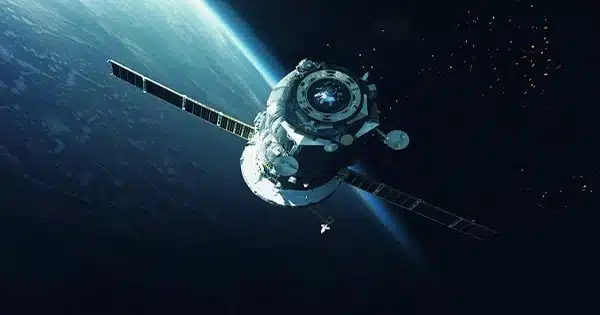
The team, all members of a JWST program led by Ilaria Pascucci (University of Arizona), wants to know how this process works so they may better comprehend its history and impact on our solar system.
“These winds could be driven either by high-energy stellar photons (the star’s light) or by the magnetic field that weaves the planet-forming disk,” Bajaj said.
Dr. Gorti of the SETI Institute has been researching disk dispersal for decades, and she and a colleague predicted the strong argon emission detected by JWST. She said that she was “excited to finally be able to disentangle the physical conditions in the wind to understand how they launch.”
Planetary systems, such as our solar system, appear to include more stony objects than gas-rich ones. The inner planets, the asteroid belt, and the Kuiper belt all orbit our sun. However, scientists have long known that planet-forming disks begin with 100 times more gas than solids, raising an important question: when and how does the majority of the gas depart the disk/system?
During the early stages of planetary system formation, planets form in a spinning disk of gas and dust surrounding the newborn star. These particles clump together to form planetesimals, which are gradually larger chunks. Over time, these planetesimals collide and bind together, eventually creating planets. Planets’ types, sizes, and locations are determined by the amount of material accessible and how long it remains in the disk. The evolution and distribution of the disk thereby determine the outcome of planet formation.
In another publication, the same group, led by Dr. Andrew Sellek of Leiden Observatory, used simulations of stellar photon dispersal to distinguish between the two. They compare the simulations to the real observations and conclude that dispersal by high-energy star photons can explain the observations and hence cannot be ruled out.
Dr. Sellek went on to explain that “the simultaneous measurement of all four lines by JWST proved crucial to pinning down the properties of the wind and helped us to demonstrate that significant amounts of gas are being dispersed.”
To put it in context, the researchers estimate that the mass dispersed each year is similar to that of the moon. The Astronomical Journal is now reviewing a companion study that will go into greater detail about these findings.
The [Ne II] line was observed towards numerous planet-forming disks in 2007 using the Spitzer Space Telescope and was quickly identified as a wind tracer by project lead Prof. Pascucci at the University of Arizona; this altered research efforts centered on disk gas dispersal. The finding of spatially resolved [Ne II] and the first detection of [Ar III] with the JWST may be the next step toward altering our knowledge of this process.
“We first used neon to study planet-forming disks more than a decade ago, testing our computational simulations against Spitzer data and new observations obtained with the ESO VLT,” explained Professor Richard Alexander of the University of Leicester School of Physics and Astronomy. We learnt a lot, but our observations did not allow us to quantify how much mass the disks were losing. The latest JWST data are magnificent, and the ability to resolve disk winds in images is something I never imagined possible. With more observations like this to come, JWST will allow us to better comprehend nascent planetary systems than ever before.”
Furthermore, the scientists determined that the inner disk of T Cha evolves on very small timescales of decades and that T Cha’s JWST spectrum differs from the earlier Spitzer spectrum. According to Chengyan Xie of the University of Arizona, the primary author of this ongoing study, this mismatch could be explained by a small, asymmetric inner disk that has lost some of its mass in only 17 years. Along with the other investigations, this suggests that T Cha’s disk is nearing the end of its evolution.
Xie goes on to say, “We might be able to witness the dispersal of all the dust mass in T Cha’s inner disk within our lifetime.”
The ramifications of these discoveries provide fresh insights into the intricate dynamics that result in the dispersal of gas and dust, which is necessary for planet formation. Understanding the principles of disk dispersal allows scientists to better forecast the timescales and circumstances conducive to planet formation. The team’s findings exhibit JWST’s capabilities and pave the way for future research into planet formation dynamics and the evolution of circumstellar disks.
The data utilized in this study were collected using the JWST/MIRI instrument under the General Observers Cycle 1 program PID 2260 (PI: I. Pascucci). The research team consists of Naman Bajaj (graduate student), Prof. Ilaria Pascucci, Dr. Uma Gorti, Prof. Richard Alexander, Dr. Andrew Sellek, Dr. Jane Morrison, Prof. Andras Gaspar, Prof. Cathie Clarke, Chengyan Xie (graduate student), Dr. Giulia Ballabio, and Dingshan Deng (graduate student).