KTH Royal Institute of Technology (KTH) researchers have developed a method of emitting light for use in quantum circuits. Quantum computing today relies on states of matter, i.e., electrons carrying qubits of information, to perform multiple calculations at the same time in a fraction of the time required by classical computing.
The promise of a quantum internet is predicated on the complexities of using light to transmit quantum information over fiber optic networks. Researchers who developed integrated chips that can generate light particles on demand reported a potential step forward today, and the promise of a quantum internet is dependent on the complexities of harnessing light to transmit quantum information over fiber optic networks. Researchers in Sweden reported today that they developed integrated chips that can generate light particles on demand and without the need for extreme refrigeration.
Quantum computing is reliant on electrons that carry qubits of information to execute different calculations at the same time, in a fraction of the time it takes conventional computers. KTH’s Ali Elshaari said the technique enables photon emitters to be precisely positioned in integrated optical circuits that convey light.
Val Zwiller, Professor at KTH Royal Institute of Technology and co-author of the study, believes that harnessing optical photons is a more promising approach for seamlessly integrating quantum computing with fiber-optic networks, which are currently used by the internet.
He claims that “the photonic approach provides a natural link between communication and computation.” “This is significant because the ultimate goal is to transmit the processed quantum information using light.”
The researchers used hexagonal boron nitride’s single-photon-emitting properties, which they combined with silicon nitride waveguides to direct the emitted photons. However, in order for photons to deliver qubits on demand in quantum systems, they must be emitted deterministically rather than probabilistically. This can be done in artificial atoms at extremely low temperatures, but a research group at KTH reported today that they have found a way to make it work in optical integrated circuits at room temperature.
According to Ali Elshaari, Associate Professor at KTH Royal Institute of Technology and co-author of the study, the new method allows photon emitters to be precisely positioned in integrated optical circuits that resemble copper wires for electricity but carry light instead.
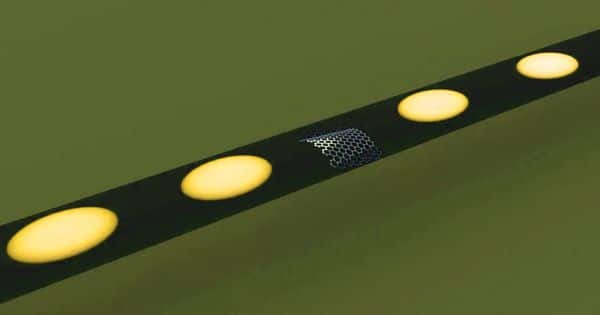
Quantum dots (QDs) are ideal sources of non-classical light, including single and entangled photons. However, the requirements for practical applications, such as integrated quantum information processing circuits, are far more stringent than most current QD systems can meet.
The single-photon-emitting properties of hexagonal boron nitride (hBN), a layered material, were exploited by the researchers. hBN is a compound that is commonly used to give self-lubricating properties to ceramics, alloys, resins, plastics, and rubbers. They combined the material with silicon nitride waveguides to direct the photons emitted.
According to Elshaari, quantum circuits with light are either operated at cryogenic temperatures (plus 4 Kelvin above absolute zero) with atom-like single-photon sources or at room temperature with random single-photon sources. The KTH technique, on the other hand, enables optical circuits with on-demand emission of light particles at room temperature.
“Unless you do a heralding measurement in existing optical circuits operating at room temperature, you never know when the single photon is generated,” Elshaari says. “We developed a deterministic process for precisely positioning light-particle emitters in an integrated photonic circuit that operates at room temperature.”
The researchers reported the coupling of an hBN single-photon emitter to silicon nitride waveguides, as well as the development of a method for imaging the quantum emitters. The team then used a hybrid approach to build the photonic circuits with respect to the quantum source locations, employing electron beam lithography and etching while maintaining the high-quality nature of the quantum light.
The achievement paves the way for hybrid integration or the incorporation of atom-like single-photon emitters into photonic platforms that are incapable of emitting light efficiently on demand.