Rice University physicists have discovered a method to capture the world’s coldest plasma in a magnetic bottle, a technological breakthrough that could advance research into clean energy, space weather, and astrophysics.
“To understand how the solar wind interacts with the Earth, or to generate clean energy from nuclear fusion, one must first understand how plasma – a soup of electrons and ions – behaves in a magnetic field,” said Rice Dean of Natural Sciences Tom Killian, the corresponding author of a study published in Physical Review Letters about the work.
Killian and graduate students Grant Gorman and MacKenzie Warrens created a plasma about 1 degree above absolute zero, or approximately -272 degrees Celsius, using laser-cooled strontium and briefly trapped it with forces from surrounding magnets. It is the first time an ultracold plasma has been magnetically confined, and Killian, who has studied ultracold plasmas for more than two decades, believes it opens the door to further research into plasmas in a variety of settings.
Physicists have discovered a way to trap the world’s coldest plasma in a magnetic bottle, a technological achievement that could advance research into clean energy, space weather and solar physics.
“This provides a clean and controllable testbed for studying neutral plasmas in far more complex environments, such as the sun’s atmosphere or white dwarf stars,” said Killian, a physics and astronomy professor. “It’s extremely beneficial to have such cold plasma and such clean laboratory systems. Beginning with a simple, small, well-controlled, well-understood system allows you to remove some of the clutter and truly isolate the phenomenon you want to observe.”
This is significant for study co-author Stephen Bradshaw, a Rice astrophysicist who studies plasma phenomena on the sun. “Throughout the sun’s atomosphere, the (strong) magnetic field has the effect of altering everything relative to what you would expect without a magnetic field, but in very subtle and complicated ways that can really trip you up if you don’t have a really good understanding,” Bradshaw, an associate professor of physics and astronomy, explained.
Solar physicists rarely get a clear view of specific features in the sun’s atmosphere because part of the atmosphere lies between the camera and those features, and unrelated phenomena in the intervening atmosphere obscure what they want to see.
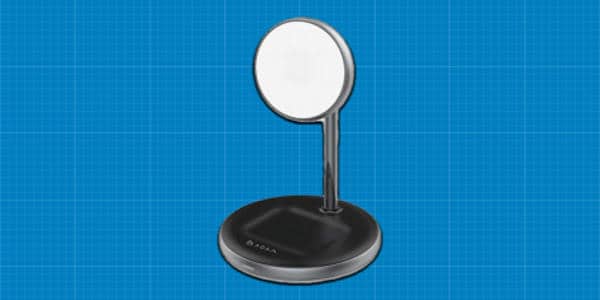
“Unfortunately, due to this line-of-sight issue, observational measurements of plasma properties are associated with quite a bit of uncertainty,” Bradshaw explained. “However, as we gain a better understanding of the phenomena and, crucially, use the laboratory results to test and calibrate our numerical models, we hope to reduce the uncertainty in these measurements.”
Plasma is one of the four fundamental states of matter, but unlike solids, liquids, and gases, plasmas are rarely encountered in everyday life because they occur in extremely hot environments such as the sun, a lightning bolt, or a candle flame. Killian’s plasmas are soups of electrons and ions, like those hot plasmas, but they’re made cold by laser-cooling, a technique developed a quarter-century ago to trap and slow matter with light.
The quadrupole magnetic setup used to trap the plasma, according to Killian, is a standard part of the ultracold setup used by his lab and others to create ultracold plasmas. However, figuring out how to trap plasma with magnets proved difficult because the magnetic field interferes with the optical system that physicists use to examine ultracold plasmas.
“Our diagnostic is laser-induced fluorescence,” he explained. “We shine a laser beam onto the ions in our plasma, and if the frequency of the beam is just right, the ions scatter photons very effectively.” “You can photograph them to see where the ions are, and you can even measure their velocity by looking at the Doppler shift, similar to how a radar gun determines how fast a car is moving. However, magnetic fields shift around the resonant frequencies, and we must separate the shifts in the spectrum caused by the magnetic field from the Doppler shifts we’re interested in observing.”
This significantly complicates experiments, and to make matters even more complicated, magnetic fields change dramatically throughout the plasma. “So we have to deal with not just a magnetic field, but a magnetic field that varies in space in a fairly complicated way in order to understand the data and figure out what’s going on in the plasma,” Killian explained. “After we got the data, we spent a year just trying to figure out what we were seeing.”
The magnetic field also complicates the plasma behavior in the experiments. That is why the trapping technique could be so beneficial. “There’s a lot of complication as our plasma expands across these field lines and begins to feel the forces and become trapped,” Killian explained. “This is a very common phenomenon, but it’s very complicated, and we need to understand it.”
Natural examples include solar wind, which is a stream of high-energy plasma from the sun that causes the aurora borealis, or northern lights. When solar wind plasma strikes Earth, it interacts with our planet’s magnetic field, and the details of those interactions remain unknown. Another example is fusion energy research, in which physicists and engineers hope to recreate the conditions inside the sun in order to produce a large amount of clean energy.
Killian claims that the quadrupole magnetic setup used by him, Gorman, and Warrens to bottle their ultracold plasmas is similar to designs developed by fusion energy researchers in the 1960s. The plasma for fusion must be approximately 150 million degrees Celsius, and magnetically containing it is a challenge, according to Bradshaw, in part due to unanswered questions about how the plasma and magnetic fields interact and influence one another.
“One of the major challenges is keeping the magnetic field stable long enough to actually contain the reaction,” Bradshaw explained. “As soon as there is a slight perturbation in the magnetic field, it expands and ‘pfft,’ the nuclear reaction is ruined.”
“You have to keep things really, really stable for it to work well,” he said. “Again, studying things in a really nice, pristine laboratory plasma could help us better understand how particles interact with the field.”