Synthetic CO2 fixation in live cells entails creating and introducing new pathways to convert carbon dioxide into organic molecules. This technique mimics natural photosynthesis but is tailored to be more efficient or to create specific desired goods. Three modules creating a novel CO2 fixation cycle have been successfully established in E.coli.
Synthetic biology provides the opportunity to create biochemical pathways for carbon dioxide (CO2) capture and conversion. The Max-Planck-Institute for Terrestrial Microbiology has created a synthetic biochemical cycle that transforms CO2 straight into the central building block Acetyl-CoA. The researchers were able to construct all three cycle modules in the bacteria E.coli, which is a significant step toward establishing synthetic CO2 fixing mechanisms in living cells.
Developing novel methods for CO2 capture and conversion is critical to addressing the climate catastrophe. Synthetic biology provides the door to building new-to-nature CO2-fixation mechanisms that absorb CO2 more efficiently than those established by nature. However, implementing those novel-to-nature mechanisms in many in vitro and in vivo systems remains a significant problem.
Tobias Erb’s group has now designed and built the THETA cycle, a new synthetic CO2-fixation process. It contains numerous important metabolites as intermediates and produces the primary building block, acetyl-CoA. This property allows it to be separated into modules and integrated into the central metabolism of E. coli.
Bringing parts of the THETA cycle into living cells is an important proof-of-principle for synthetic biology. Such modularization of this cycle in E. coli opens the door to the realization of highly complex, orthogonal new-to-nature CO2-fixation pathways in cell factories.
Tobias Erb
The complete THETA cycle is made up of 17 biocatalysts and is based on the two fastest CO2-fixing enzymes known to date: crotonyl-CoA carboxylase/reductase and phosphoenolpyruvate carboxylase. These potent biocatalysts were discovered in bacteria by the researchers. Despite the fact that each of the carboxylases can collect CO2 more than ten times faster than RubisCO, the CO2-fixing enzyme in chloroplasts, evolution has yet to bring these powerful enzymes together in natural photosynthesis.
In one cycle, the THETA cycle transforms two CO2 molecules into one acetyl-CoA molecule. Acetyl-CoA is a key metabolite in practically all cellular metabolism and a building block for a diverse range of essential biomolecules such as biofuels, biomaterials, and medicines, making it a substance of great interest in biotechnological applications. The researchers were able to establish the cycle’s functionality after building it in test tubes. The training then began: the team was able to improve the acetyl-CoA yield by a factor of 100 through rational and machine learning-guided optimization over numerous rounds of experimentation.
In order to test its in vivo feasibility, incorporation into the living cell should be carried out step by step. To this end, the researchers divided the THETA cycle into three modules, each of which was successfully implemented into the bacterium E. coli. The functionality of these modules was verified through growth-coupled selection and/or isotopic labelling.
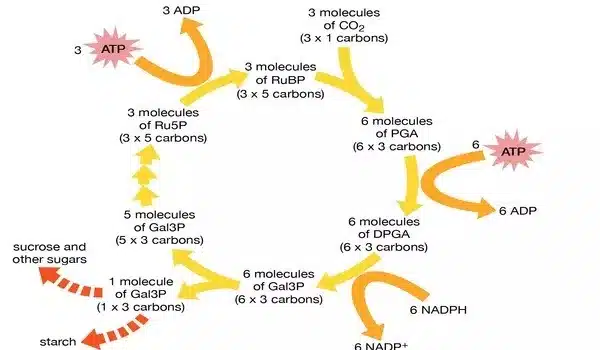
“What makes this cycle unique is that it contains several intermediates that serve as key metabolites in the bacterium’s metabolism. This overlap provides an opportunity to build a modular strategy for its implementation,” adds Shanshan Luo, the study’s principal author. “In E. coli, we were able to demonstrate the functionality of the three individual modules. However, we have yet to complete the entire cycle so that E. coli can grow entirely on CO2,” she says.
Closing the THETA cycle remains a huge difficulty since all 17 reactions must be timed with E. coli’s natural metabolism, which comprises hundreds to thousands of events. The researcher underlines that displaying the entire cycle in vivo is not the main goal. “Our cycle has the potential to become a versatile platform for producing valuable compounds directly from CO2 through extending its output molecule, acetyl-CoA.” According to Shanshan Luo,
“Bringing parts of the THETA cycle into living cells is an important proof-of-principle for synthetic biology,” says Tobias Erb. “Such modularization of this cycle in E. coli opens the door to the realization of highly complex, orthogonal new-to-nature CO2-fixation pathways in cell factories.” We’re learning how to fundamentally rewire the cellular metabolism in order to construct a synthetic autotrophic operating system for the cell.”