Water is possibly the most important natural resource on the planet. Scientists are pursuing more innovative ways to use and reuse existing water, as well as designing new materials to improve water purification methods, in response to rising demand and increasingly depleted water resources. However, existing knowledge gaps are limiting transformative advances in membrane technology. Synthetically created semi-permeable polymer membranes used for contaminant solute removal can provide a level of advanced treatment and improve the energy efficiency of treating water; however, existing knowledge gaps are limiting transformative advances in membrane technology. One basic problem is learning how the affinity, or the attraction, between solutes and membrane surfaces impacts many aspects of the water purification process.
Researchers explain the relevance of macroscopic characterizations of solute-to-surface affinity. Researchers’ computational methods pave the way for next-generation membrane technology for water purification.
“Fouling — where solutes stick to and gunk up membranes — significantly reduces performance and is a major impediment in designing membranes to treat produced water,” said M. Scott Shell, a chemical engineering professor at UC Santa Barbara who studies soft materials and biomaterials through computational simulations. “If we can fundamentally understand how the chemical composition of membrane surfaces affects solute stickiness, including possible patterning of functional groups on these surfaces, we can begin to design next-generation, fouling-resistant membranes that repel a wide range of solute types.”
Shell and lead author Jacob Monroe, a recent Ph.D. graduate of the department and a former member of Shell’s research group, explain the importance of macroscopic characterizations of solute-to-surface affinity in a paper published in the Proceedings of the National Academy of Sciences (PNAS).
“Solute-surface interactions in water determine the behavior of a wide range of physical phenomena and technologies, but they are especially important in water separation and purification, where many distinct types of solutes must often be removed or captured,” explained Monroe, who is now a postdoctoral researcher at the National Institute of Standards and Technology (NIST).
“This work addresses the grand challenge of understanding how to design next-generation membranes capable of handling massive yearly volumes of highly contaminated water sources, such as those produced in oilfield operations, where solute concentrations are high and chemistries are diverse.”
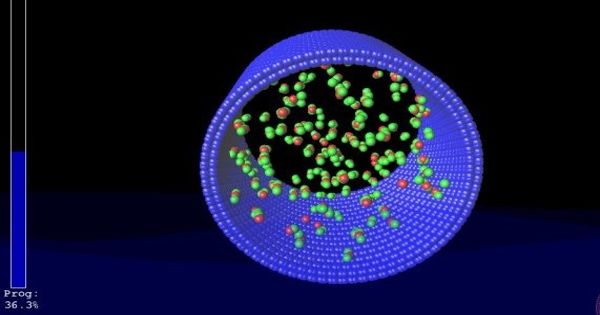
Solutes are frequently classified as hydrophilic, which means they like water and dissolve easily in it, to hydrophobic, which means they dislike water and prefer to separate from it, such as oil. Water beads up on hydrophobic surfaces and spreads out on hydrophilic surfaces; for example, water beads up on hydrophobic surfaces and spreads out on hydrophilic surfaces. Hydrophilic solutes prefer hydrophilic surfaces, while hydrophobic solutes prefer hydrophobic surfaces. The researchers confirmed the expectation that “like sticks to like,” but they also discovered, surprisingly, that the full picture is more complex.
“Among the wide range of chemistries that we considered, we discovered that hydrophilic solutes like hydrophobic surfaces, and that hydrophobic solutes like hydrophilic surfaces, though these attractions are weaker than those of like to like,” Monroe explained, referring to the eight solutes tested, which ranged from ammonia and boric acid to isopropanol and methane. To provide a fundamental perspective on solute-surface affinity, the group chose small-molecule solutes commonly found in produced waters.
The computational research group created an algorithm for repatterning surfaces by rearranging surface chemical groups to minimize or maximize the affinity of a given solute to the surface, or to maximize the surface affinity of one solute relative to that of another. The method was based on a genetic algorithm that “evolved” surface patterns in a manner similar to natural selection, optimizing them toward a specific function goal.
The team discovered through simulations that surface affinity was poorly correlated to traditional methods of solute hydrophobicity, such as how soluble a solute is in water. Instead, they discovered a stronger link between surface affinity and the way water molecules change their structures in response to being near a surface or a solute. In some cases, these neighboring waters were forced to adopt unfavorable structures; solutes could then reduce the number of such unfavorable water molecules by moving closer to hydrophobic surfaces, providing an overall driving force for affinity.
“The missing piece was understanding how water molecules near a surface are structured and move around it,” Monroe explained. “When compared to bulk water or water far away from the surface, water structural fluctuations are enhanced near hydrophobic surfaces. We discovered that fluctuations influenced the stickiness of every small solute type we tested.
The discovery is significant because it demonstrates that when developing new surfaces, researchers should focus on the response of water molecules around them rather than being guided by conventional hydrophobicity metrics.
According to Monroe and Shell’s findings, surfaces composed of various molecular chemistries may be the key to achieving multiple performance goals, such as preventing a variety of solutes from fouling a membrane.
“Surfaces with a variety of chemical groups have a lot of potential. “We demonstrated that the presence of different surface groups, as well as their arrangement or pattern, influence solute-surface affinity,” Monroe said. “By simply rearranging the spatial pattern, it is now possible to significantly increase or decrease the surface affinity of a given solute without changing the number of surface groups present.”
According to the team, their findings show that computational methods can contribute in significant ways to next-generation membrane systems for sustainable water treatment. Shell, the John E. Myers Founder’s Chair in Chemical Engineering, said, “This work provided detailed insight into the molecular-scale interactions that control solute-surface affinity.” “Moreover, it demonstrates that surface patterning provides a powerful design strategy in engineering membranes that are resistant to fouling by a variety of contaminants and can precisely control how each solute type is separated out.” As a result, it provides molecular design rules and targets for next-generation membrane systems capable of purifying highly contaminated waters while conserving energy.”
The majority of the surfaces examined were simplified model systems designed to aid in analysis and comprehension. According to the researchers, the natural next step will be to investigate increasingly complex and realistic surfaces that more closely resemble actual membranes used in water treatment. Another important step toward bringing the modeling closer to membrane design will be to go beyond simply understanding how sticky a membrane is for a solute and instead compute the rates at which solutes move through membranes.