Detecting bacteria and viruses with fluorescent nanotubes is a rapidly evolving area of nanotechnology and biosensing research. Fluorescent nanotubes, particularly carbon nanotubes (CNTs), can be engineered to have fluorescent properties, making them useful for detecting and identifying microorganisms such as bacteria and viruses.
The new carbon nanotube sensor design is similar to a molecular toolbox, which can be used to quickly assemble sensors for a variety of purposes, such as detecting bacteria and viruses.
An interdisciplinary research team from Bochum, Duisburg, and Zurich has developed a new method for building modular optical sensors capable of detecting viruses and bacteria. The researchers used fluorescent carbon nanotubes in conjunction with a novel type of DNA anchor that acts as a molecular handle for this purpose. The anchor structures can be used to attach biological recognition units to the nanotubes, such as antibodies and aptamers. Following that, the recognition unit can interact with bacterial or viral molecules to the nanotubes. These interactions have an impact on the fluorescence of the nanotubes, increasing or decreasing their brightness.
A team consisting of Professor Sebastian Kruss, Justus Metternich and four co-workers from Ruhr University Bochum (Germany), the Fraunhofer Institute for Microelectronic Circuits and Systems and the ETH Zurich reported their findings in the Journal of the American Chemical Society.
This is advantageous if you consider measurements beyond simple aqueous solutions. We need to measure in complex environments, such as cells, blood, or the organism itself, for diagnostic applications.
Sebastian Kruss
Straightforward customisation of carbon nanotube biosensors
The researchers used tubular carbon nanosensors with a diameter of less than one nanometre. When exposed to visible light, carbon nanotubes emit light in the near-infrared range. Near-infrared light is not visible to the naked eye. However, because the level of other signals in this range is so low, it is ideal for optical applications. Sebastian Kruss’ team had previously demonstrated how to manipulate the fluorescence of nanotubes in order to detect vital biomolecules. The researchers were now looking for a simple way to customize the carbon sensors for use with different target molecules.
The key to success was the use of DNA structures with guanine quantum defects. This entailed connecting DNA bases to the nanotube in order to create a defect in the nanotube’s crystal structure. As a result, the nanotubes’ fluorescence changed at the quantum level. Furthermore, the defect served as a molecular handle, allowing the introduction of a detection unit that can be tailored to the specific target molecule in order to identify a specific viral or bacterial protein.
“Through the attachment of the detection unit to the DNA anchors, the assembly of such a sensor resembles a system of building blocks – except that the individual parts are 100,000 times smaller than a human hair,” outlines Sebastian Kruss.
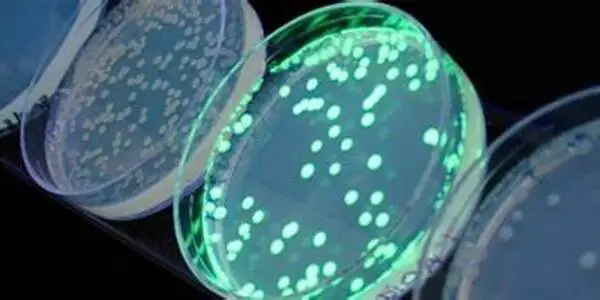
Sensor identifies different bacterial and viral targets
The group showcased the new sensor concept using the SARS CoV-2 spike protein as an example. To this end, the researchers used aptamers, that bind to the SARS CoV-2 spike protein. “Aptamers are folded DNA or RNA strands. Due to their structure, they can selectively bind to proteins,” explains Justus Metternich. “In the next step, one could transfer the concept to antibodies or other detection units.”
The fluorescent sensors reliably detected the presence of the SARS-CoV-2 protein. The selectivity of sensors with guanine quantum defects was higher than the selectivity of sensors without such defects. Furthermore, the sensors with guanine quantum defects were more stable in solution.
“This is advantageous if you consider measurements beyond simple aqueous solutions. We need to measure in complex environments, such as cells, blood, or the organism itself, for diagnostic applications,” says Sebastian Kruss, who heads the Functional Interfaces and Biosystems Group at Ruhr University Bochum and is a member of the Ruhr Explores Solvation Cluster of Excellence (RESOLV) and the International Graduate School of Neuroscience.