Nuclear clocks could enable scientists to test humanity’s basic knowledge of how reality functions, even though they are not primarily helpful in telling the time.
Author Caleb Davies Thorsten Schumm is a clockmaker, but not the kind that works at a table covered in springs and gears while wearing a magnifying loupe in one eye. No, he is producing a watch that is in a completely distinct class.
Atomic clocks may seem common enough, but if Schumm’s study is successful, a nuclear clock might be created. In addition to more than just telling the time, it might be able to unlock some of the universe’s most tightly held mysteries.
Professor Schumm of the Vienna University of Technology in Austria said, “This is still a fantasy.” No one is aware of how to do it.
He wants to alter that and, in the process, reveal some of the basic natural forces.
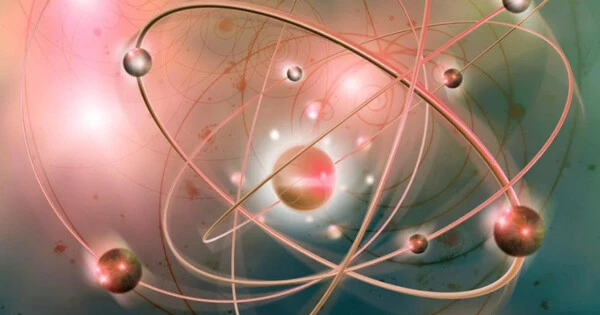
Split second: Anything that oscillates at predictable periods and can be read can be the foundation of a clock. Mechanical timepieces were the first. Today, a quartz crystal’s electromechanical vibrations are used by many wristwatches.
But with the introduction of atomic timepieces in the 1950s, clock technology advanced significantly.
A nucleus and an orbiting swarm of electrons make up an atom. The “quantum shifts” that these electrons undergo determine the rate at which an atomic clock ticks.
This is how it functions. A stream of energy can be absorbed by an electron, causing it to transition from its lower energy “ground state” to a higher energy “excited state.” Then, as they return to the ground condition, they can let go of that energy packet.
These energy changes take place at a specific frequency that can be used to maintain time. Everything occurs incredibly quickly.
A cesium-133 particle is excited by an energy packet that oscillates 9 192 631 770 times in a second, for example. Atomic clocks generate a huge number of vibrations, or ticks, which is why they are so accurate. Therefore, even if the readout mechanism misses one or two of them when there are more than 9 billion per second, it usually won’t cause too much of an issue.
The nuclear timepiece is unique. The tick would be driven by the movements of the nucleus itself, not by electrons. These move much more quickly than electron transition pulses do.
However, as Schumm notes, efforts are still being made to set up a nuclear timepiece.
Happy coincidence: In part due to coincidence, he became interested in solving this nuclear riddle.
It turns out that the most straightforward substance from which a nuclear clock could be constructed is an uncommon isotope of the element thorium-229. This is due to the fact that it is believed to have the fastest ticks of any nucleus. Additionally, Schumm’s workplace is one of the few institutions with access to this information.
There is no native source of thorium-229. It can only be created through the nuclear decay of specific uranium kinds. The Vienna University of Technology is able to acquire some thorium-229 from uranium leftover from long-ago nuclear tests thanks to a collaboration with Oakridge National Laboratory in the US. Schumm was aware that both the name of the element and his first name were derived from the legendary Norse deity Thor. That made me chuckle, he said.
It’s high time: Schumm has been working on the ThoriumNuclearClock project, which is financed by the EU and runs until the beginning of 2026, since 2020.
Along with Marianna Safronova from the University of Delaware in the US and Peter Thirolf from LMU Munich in Germany, they are the project’s main investigators, along with their colleague Professor Ekkehard Peik of Germany’s National Metrology Institute in Braunschweig.
A laser that is precisely calibrated to the appropriate energy level must be used to start a nuclear clock ticking. However, the energy frequency needed for the majority of atoms is far beyond what can be achieved with available laser technology.
One of the biggest stable atoms known to man is thorium-229. Although no one really knows how or why it does this, it was once believed that it could adopt a state with a very low energy that current lasers could achieve.
It wasn’t initially entirely obvious that this form of thorium-229 even existed, according to Schumm.
It is now widely acknowledged to exist. Schumm and his associates released their analysis of the isotope’s energy level in 2020. They have since proceeded to add to their understanding. All of that makes it possible to actually test the timepiece. Schumm and his colleagues have been developing a laser that is specifically made to stimulate thorium at the ideal frequency. They will soon aim this laser at some confined thorium atoms in an effort to ignite their ticking.
Peik said, “This experiment is something that has never been done before, so we are very enthusiastic about the results.” We and others have attempted similar thorium-229 experiments in the past without results. We believe we are much better equipped this time.
Crystal clear: The thorium atoms will be held in atomic traps for those tests, which is a very delicate process. Schumm therefore oversaw a two-year EU-funded project dubbed CRYSTALCLOCK that sought to create a more straightforward design and readout mechanism for a nuclear clock while ThoriumNuclearClock was already underway.
The concept behind this experiment was to grow a calcium fluoride crystal while dispersing thorium-229 atoms throughout it. This results in a solid substance that is much more manageable than atomic traps.
In a paper they released, Schumm and his associates—among them, Dr. Tomas Sikorsky—proved that these thorium-doped crystals could be grown in 2022. The process of figuring out how to interpret these crystals’ ticks will come next.
According to Schumm, using nuclear tomography could be modified for this purpose and would be a lot simpler than using thorium atoms in traps.
Forces of nature: This is important because it allows us to verify our basic assumptions about how reality functions rather than because we need more accurate clocks.
The four basic forces of the universe are gravity, electromagnetism, the weak nuclear force, and the strong nuclear force, according to the best theories of physics. These numbers are frequently referred to as “constants” because of how powerful these factors are known to be.
However, it is unknown if the power of these factors has always been the same or ever will be. There are hints that the forces were more powerful in the distant past, near to the Big Bang, and they might even still be changing slightly today.
Putting that to the test might be feasible with the help of atomic and nuclear clocks. Since electromagnetism has a strong influence on how quickly an atomic clock ticks, a shift in tick speed would indicate a drift in the underlying force.
Atomic clocks, despite their astounding accuracy, may never be able to detect any change to electromagnetism because it is such a weak force.
The strong force, in comparison, affects the rate at which a nuclear clock ticks. Therefore, if and when a functional nuclear clock were to be developed, it could be used to track any long-term alterations to the strong force.
It’s not about having a better clock when you move from atoms to nuclei, according to Schumm. The first nuclear clock may not even be as accurate as the finest atomic clocks. The key is having an entirely new technology that could essentially measure the strong force.