An international team of physicists led by the University of Minnesota discovered that when used as a very thin layer, a unique superconducting metal is more resilient. The study is the first step toward a larger goal of understanding unusual superconducting states in materials, which may one day be used in quantum computing.
Four faculty members from the University of Minnesota’s School of Physics and Astronomy are involved in the collaboration, including Associate Professor Vlad Pribiag, Professor Rafael Fernandes, and Assistant Professors Fiona Burnell and Ke Wang, as well as physicists from Cornell University and other institutions. The research was published in Nature Physics, a peer-reviewed scientific journal published monthly by Nature Research.
Niobium diselenide (NbSe2) is a superconducting metal, which means it can conduct electricity or transport electrons between atoms with no resistance. It is common for materials to behave differently at very small sizes, but NbSe2 has potentially beneficial properties. The researchers discovered that the material in 2D form (a very thin substrate only a few atomic layers thick) is a more resilient superconductor than thicker samples of the same material because it has a two-fold symmetry.
A study led by physics researchers has discovered that a unique superconducting metal is more resilient when used as a very thin layer.
Pribiag and Wang began investigating atomically thin 2D superconducting devices after being inspired by Fernandes and Burnell’s theoretical prediction of exotic superconductivity in this 2D material. “Like a snowflake, we expected it to have a six-fold rotational pattern,” Wang stated. “Despite having a six-fold structure, it only demonstrated two-fold behavior in the experiment.”
“This was one of the first times [this phenomenon] was seen in a real material,” Pribiag said.
The newly discovered two-fold rotational symmetry of the superconducting state in NbSe2 was attributed to the mixing of two closely competing types of superconductivity, namely the conventional s-wave type, which is typical of bulk NbSe2, and an unconventional d- or p-type mechanism, which emerges in few-layer NbSe2. In this system, the energies of the two types of superconductivity are very similar. As a result, they interact and compete with one another.
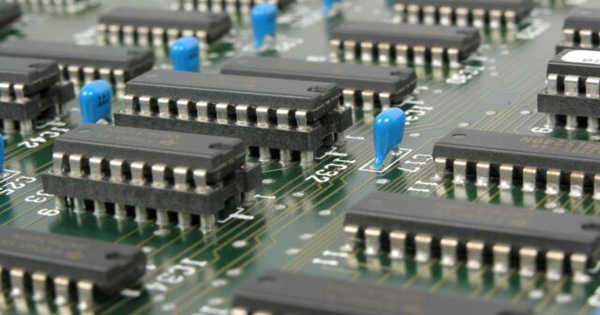
According to Pribiag and Wang, later discovered that physicists at Cornell University were reviewing the same physics using a different experimental technique, quantum tunneling measurements. They decided to publish a comprehensive study by combining their findings with the Cornell research.
Burnell, Pribiag, and Wang intend to expand on these preliminary findings by studying the properties of atomically thin NbSe2 in combination with other exotic 2D materials, which could eventually lead to the use of unconventional superconducting states, such as topological superconductivity, to build quantum computers.
“What we want is an atomically flat interface,” Pribiag explained. “We believe that this system will provide us with a better platform for studying materials in order to use them in quantum computing applications.”
According to Nandini Trivedi, a physicist at Ohio State University who was not involved in the research, the electronic “flatlands” discovered by these measurements are promising test beds for theories of superconductivity. She investigates instances in which electrons in ultrathin superconductors form a swarm of tight-knit particle islands. Members of Manoharan’s team observed their electrons self-organizing in the same way, and with more of this distinct behavior than seen in materials that scientists purposefully engineer as flat.
The research at the University of Minnesota was primarily funded by the National Science Foundation (NSF) through the University of Minnesota Materials Research Science and Engineering Center (MRSEC). The research at Cornell was funded by the Office of Naval Research (ONR) and the National Science Foundation (NSF). The Swiss National Science Foundation funded the research in Switzerland.