Atomic nuclei are intricate structures made up of protons and neutrons that are held together by the strong nuclear force. The collective motion of these protons and neutrons within the nucleus is referred to as “vibrating” atomic nuclei. The shell model and the liquid drop model, which provide different perspectives on the behavior of atomic nuclei, can be used to describe this motion.
A group of physicists led by Professor Stephan Schiller Ph.D. from Heinrich Heine University Düsseldorf (HHU) measured the wave-like vibration of atomic nuclei with unprecedented precision using ultra-high-precision laser spectroscopy on a simple molecule. The physicists report in the scientific journal Nature Physics that they can now confirm the wave-like movement of nuclear material with greater precision than ever before and that they have found no evidence of any deviation from the established force between atomic nuclei.
For nearly a century, simple atoms have been the subject of precise experimental and theoretical investigations, with pioneering work on the description and measurement of the hydrogen atom, the simplest atom with only one electron. At the moment, the energies of hydrogen atoms – and thus their electromagnetic spectrum – are the most precisely computed energies of a bound quantum system. Because extremely precise measurements of the spectrum are possible, comparing theoretical predictions and measurements allows testing of the theory on which the prediction is based.
Such tests are very important. Researchers around the world are seeking – albeit unsuccessfully to date – evidence of new physical effects that could occur as a result of the existence of Dark Matter. These effects would lead to a discrepancy between measurement and prediction.
The experimentally determined transition frequency and the theoretical prediction agree. In conjunction with previous findings, we have established the most precise test of charged baryon quantum motion: Any deviation from the established quantum laws, if it exists at all, must be less than 1 part in 100 billion.
Professor Schiller
For a long time, the simplest molecule, unlike the hydrogen atom, was not subject to precision measurements. However, a research group led by Professor Stephan Schiller Ph.D. of HHU’s Chair of Experimental Physics has devoted itself to this topic. In Düsseldorf, the group pioneered research and developed experimental techniques that are among the most precise in the world.
The molecular hydrogen ion (MHI) is the simplest molecule: a hydrogen molecule that lacks an electron and consists of three particles. H2+ is made up of two protons and an electron, whereas HD+ is made up of a proton, a deuteron (a heavier hydrogen isotope), and an electron. Protons and deuterons are charged “baryons,” or particles that are affected by the strong force.
Within the molecules, the components can behave in various ways: The electrons move around the atomic nuclei, while the atomic nuclei vibrate against or rotate around each other, with the particles acting like waves. These wave motions are described in detail by quantum theory.
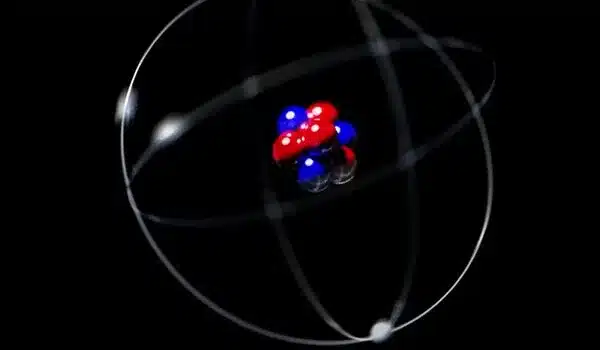
The different modes of motion determine the spectra of the molecules, which are reflected in different spectral lines. The spectra arise in a similar way to atom spectra, but are significantly more complex.
The art of modern physics research now entails measuring the wavelengths of spectral lines extremely precisely and calculating these wavelengths extremely precisely using quantum theory. A match between the two results is interpreted as proof of the predictions’ accuracy, whereas a mismatch may be a hint for “new Physics.”
The HHU physics team has refined the MHI laser spectroscopy over the years, developing techniques that have improved the experimental resolution of the spectra by multiple orders of magnitude. Their goal is to measure spectra as precisely as possible so that theoretical predictions can be tested. This enables the identification of any potential deviations from the theory and thus also starting points for how the theory might need to be modified.
Professor Schiller’s team has improved experimental precision to a level better than theory. To achieve this, the physicists in Düsseldorf confine a moderate number of around 100 MHI in an ion trap in an ultra-high vacuum container, using laser cooling techniques to cool the ions down to a temperature of 1 milli kelvin. This enables extremely precise measurement of the molecular spectra of rotational and vibrational transitions. Following earlier investigations of spectral lines with wavelengths of 230 ?m and 5.1 ?m, the authors now present measurements for a spectral line with the significantly shorter wavelength of 1.1 ?m in Nature Physics.
“The experimentally determined transition frequency and the theoretical prediction agree,” says Professor Schiller. In conjunction with previous findings, we have established the most precise test of charged baryon quantum motion: Any deviation from the established quantum laws, if it exists at all, must be less than 1 part in 100 billion.”
The outcome can also be interpreted in another way: A fundamental force other than the well-known Coulomb force (the force between electrically charged particles) could theoretically exist between the proton and deuteron. “Such a hypothetical force may exist in connection with the phenomenon of Dark Matter,” says lead author Dr. Soroosh Alighanbari. We have not found any evidence for such a force in the course of our measurements, but we will continue our search.”