When materials knock incredibly small sizes, odd things tend to happen. One such occurrence is the development of mesocrystals. While being composed of different individual crystals, the mesocrystals come together to create a larger, fused structure that functions like a pure, single crystal. However, these processes take place at sizes much too small for the human eye to view, and their development is incredibly difficult to witness. Owing to these difficulties, scientists have not been able to confirm precisely how mesocrystals form.
Researchers used advanced microscopy techniques to watch mesocrystals form in real-time. They used advanced transmission electron microscopy (TEM) techniques to see mesocrystals form in solution in real-time.
New study by the Pacific Northwest National Laboratory (PNNL)-led team has now used modern transmission electron microscopy (TEM) techniques to see mesocristals forming in real-time solutions. What they saw runs counter to traditional wisdom, and their findings may one day help scientists design energy storage materials and explain how minerals form in the soil.
Rather than nucleating individual crystals, beginning with crystal forming, and then arbitrarily aggregating into mesocrystals in two unrelated stages, the researchers found that nucleation and attachment were tightly coupled to create these extremely standardized structures.
“Our findings identify an important new pathway of crystallization by particle attachment and resolve key questions about mesocrystal formation,” said PNNL and University of Washington Materials Scientist Guomin Zhu. He was part of a research team led by Jim De Yoreo, PNNL Materials Scientist and co-director of the Northwest Institute for Material Physics, Chemistry and Technology. “We suspect this is a widespread phenomenon with significant implications both for the synthesis of designed nanomaterials and for understanding natural mineralization,” Zhu said.
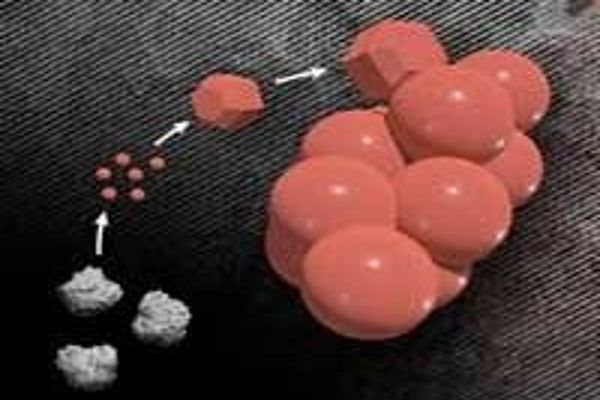
Seeing crystallization in real-time
The project took years to finish and required a large solution to the problems. For microscopy studies, the scientific team picked a model system that included hematite, an iron compound typically found in the Earth’s crust, and oxalate, a naturally abundant soil compound.
They visualized the process using in situ TEM, which allows researchers the opportunity to see the nanometer scale crystallization as it occurs. They combined this real-time method with the “freeze-and-look” TEM that allowed them to follow an individual crystal at different points during development. Theoretical calculations helped to complete the image, enabling the PNNL team to work together on how the mesocrystals formed.
Researchers typically perform most in situ TEM tests at room temperature in order to optimize the experimental setup and reduce the risk for damage to the sensitive instrument, but the mesocristal formation happens rapidly enough to be detected at about 80°C.
“The additional equipment used to heat the samples made the experiments extremely challenging, but we knew the data would be key to understanding how the mesocrystals were forming,” Zhu said. When hot, the new hematite nanocrystals make it easier for them to fasten together, which, on average, leads to final mesocristals of about the same size and shape.
Mesocrystals in nature
The chemical key to this quick, stable attachment is the oxalate molecules present in the solution. After the first few small crystals are formed, the oxalate additives help to create a chemical gradient at the interface between the liquid and the rising crystal. More chemical components required for the near-crystal nucleation of particles, which significantly increases the probability that new particles will develop in the vicinity of current ones.
Although this crystal growth mechanism has been observed under regulated conditions at very small scales, it is also likely to occur in natural systems, according to researchers. Few mineral deposits, including the Australian hematite formation, contain mesocristals. Given the natural abundance of oxalate and the discovery by the PNNL team that hematite can become mesocrystals at temperatures as low as 40 °C, it seems probable that this route of forming exists in nature.
Since mesocristals are present in nature, results can be extended to the understanding of nutrient cycles in the environment, among other applications. In addition, the approach to the development of near-uniform complex structures involves an understanding of how the processes for the formation of such materials work and how to regulate them. This work, funded by the U.S. Department of Energy, Office of Chemistry, Office of Basic Energy Sciences, Division of Chemical Sciences, Geosciences and Biosciences, is opening up new possibilities for deliberately making mesocristals or mesocristal-like materials.