For the first time, a collaboration of experimentalists from the Max Planck Institute of Quantum Optics (MPQ) and theorists at the Chinese Academy of Sciences (CAS) has successfully populated and stabilized a new type of molecule, known as field-linked tetratomic molecules. These “supermolecules” are so fragile that they can only live at extremely low temperatures. Their existence had long been hypothesized, but it had never been proven experimentally—until now.
The polyatomic molecules developed in this new study include more than two atoms and have been effectively cooled to 134 nanokelvin, which is more than 3,000 times colder than the temperature of previously created tetratomic molecules. This achievement is not only a remarkable feat in molecular physics, but it also represents a substantial advancement in the study of strange ultracold materials. The findings are reported in Nature.
About two decades ago, American theoretical physicist John Bohn and his colleagues predicted a novel type of binding between polar molecules: If the molecules have an asymmetrically distributed charge—what physicists call polarity—they can combine in an electric field to form weakly bound “supermolecules.”
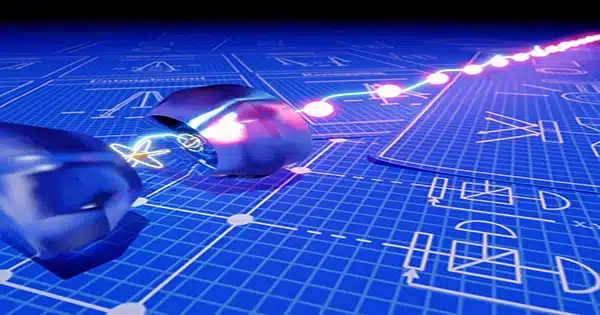
These polar molecules behave like compass needles inside a rigid shell. When compass needles are brought close together, they have a stronger pull than the Earth’s magnetic field and point toward each other rather than north.
A similar phenomenon may be observed with polar molecules, which, under certain conditions, can establish a distinct bound state through electrical forces. Their bond is reminiscent of a dancing couple hugging each other firmly but maintaining a certain space.
The bonded state of supermolecules is significantly weaker than ordinary chemical bonds, but it also has a far greater range. Supermolecules have bond lengths that are hundreds of times longer than usually bound molecules.
Because of their long-range nature, such supermolecules are extremely sensitive: even minor changes in the electric field parameters at a critical value cause huge changes in the forces between the molecules, a phenomenon known as “field-linked resonance.” This allows the researchers to control the form and size of the molecules using a microwave field.
A play with three parts: From diatomic to tetratomic molecules.
Ultracold polyatomic molecules have a complex internal structure, which opens up fascinating new possibilities in cold chemistry, precision measurements, and quantum information processing. However, their tremendous complexity in comparison to diatomic molecules makes it difficult to use traditional cooling techniques such as direct laser cooling and evaporative cooling.
Researchers in MPQ’s “NaK Lab” (sodium potassium lab), directed by Dr. Xin-Yu Luo, Dr. Timon Hilker, and Prof. Immanuel Bloch, have made a succession of pioneering and Nature-published discoveries in recent years, which were critical to finally overcoming this problem.
First, in 2021, experts in this lab developed a revolutionary chilling technique for polar molecules utilizing a high-power rotating microwave field, setting a new low-temperature record of 21 billionths of a degree above absolute zero at minus 273.15 degrees Celsius.
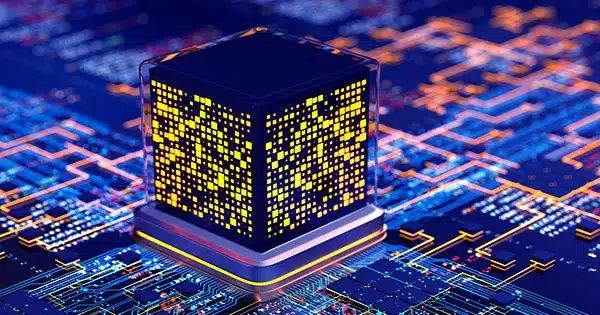
A year later, the researchers were able to create the circumstances required to observe the binding signal between these molecules in scattering tests for the first time. This gave the first indirect evidence that these novel constructions, which had been expected theoretically for a long time, exist.
Now there is concrete evidence, as the researchers were able to generate and stabilize these supermolecules during their experiment. Imaging of these “supermolecules” revealed their p-wave symmetry, a distinguishing property required for the production of topological quantum materials and potentially relevant for fault-tolerant quantum computation.
“This research will have immediate and far-reaching implications,” says Xing-Yan Chen, Ph.D. candidate and the paper’s first author. “Because the approach applies to a wide range of molecular species, it allows for a far broader exploration of ultracold polyatomic molecules. In the future, it may be possible to produce much larger and longer-living molecules, which would be particularly useful for precision metrology or quantum chemistry.
“We arrived at these findings thanks also to our close collaboration with Prof. Tao Shi and his team from the CAS,” adds Dr. Luo, the experiment’s primary investigator. “Our next goal is to cool these bosonic ‘ supermolecules’ to generate a Bose-Einstein condensate (BEC), in which the molecules move collectively. This hypothesis has significant implications for our basic understanding of quantum physics. What’s more astonishing is that by merely tweaking a microwave field, a BEC of supermolecules can convert into a novel quantum fluid of fermionic molecules while retaining the unique p-wave symmetry.”